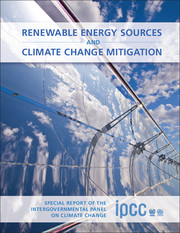
Book contents
- Frontmatter
- Contents
- Section I
- Section II
- Section III
- Chapter 1 Renewable Energy and Climate Change
- Chapter 2 Bioenergy
- Chapter 3 Direct Solar Energy
- Chapter 4 Geothermal Energy
- Chapter 5 Hydropower
- Chapter 6 Ocean Energy
- Chapter 7 Wind Energy
- Chapter 8 Integration of Renewable Energy into Present and Future Energy Systems
- Chapter 9 Renewable Energy in the Context of Sustainable Development
- Chapter 10 Mitigation Potential and Costs
- Chapter 11 Policy, Financing and Implementation
- Section IV
- Index
- References
Chapter 10 - Mitigation Potential and Costs
Published online by Cambridge University Press: 05 December 2011
- Frontmatter
- Contents
- Section I
- Section II
- Section III
- Chapter 1 Renewable Energy and Climate Change
- Chapter 2 Bioenergy
- Chapter 3 Direct Solar Energy
- Chapter 4 Geothermal Energy
- Chapter 5 Hydropower
- Chapter 6 Ocean Energy
- Chapter 7 Wind Energy
- Chapter 8 Integration of Renewable Energy into Present and Future Energy Systems
- Chapter 9 Renewable Energy in the Context of Sustainable Development
- Chapter 10 Mitigation Potential and Costs
- Chapter 11 Policy, Financing and Implementation
- Section IV
- Index
- References
Summary
Executive Summary
Renewable energy (RE) has the potential to play an important and increasing role in achieving ambitious climate mitigation targets. Many RE technologies are increasingly becoming market competitive, although some innovative RE technologies are not yet mature, economic alternatives to non-RE technologies. However, assessing the future role of RE requires not only consideration of the cost and performance of RE technologies, but also an integrative perspective that takes into account the interactions between various forces and the overall systems behaviours.
An increasing number of integrated scenario analyses are available in the published literature. They are able to provide relevant insights into the potential contribution of RE to future energy supplies and climate change mitigation. A review of 164 scenarios from 16 different large-scale integrated models was conducted through an open call. Although a collection of scenarios from the literature does not represent a truly random sample suitable for rigorous statistical analysis, a scenario overview can provide some critical and strategic insights about the role of RE in climate mitigation, in spite of the uncertainties involved.
Although it is not possible to precisely link long-term climate goals and global RE deployment levels, RE deployment significantly increases in the scenarios with ambitious greenhouse gas (GHG) concentration stabilization levels. Ambitious GHG concentration stabilization levels lead on average to higher RE deployment compared to the baseline. However, for any given long-term GHG concentration goal, the scenarios exhibit a wide range of RE deployment levels.
- Type
- Chapter
- Information
- Renewable Energy Sources and Climate Change MitigationSpecial Report of the Intergovernmental Panel on Climate Change, pp. 791 - 864Publisher: Cambridge University PressPrint publication year: 2011
References
- 36
- Cited by