Significant outcomes
-
Cannabidiol (CBD) reduced the mortality rate, decreased dopaminergic neuronal loss in the SNpc and attenuated neuroinflammation in 6-OHDA-lesioned rats
-
CBD prevented memory impairments and attenuated despair-like behaviours that were induced by bilateral intranigral 6-OHDA lesions.
-
CBD favoured the neuronal maturation of newborn neurons in the hippocampus 6-OHDA-lesioned rats.
Limitations
-
The 6-OHDA toxin model does not mimic the progressive dopaminergic loss and lacks Lewy-related pathology seen in Parkinson’s disease (PD).
-
CBD was given after intra-nigral 6-OHDA infusion. Using genetic models, for example, CBD treatment could be started before the onset of the symptoms to mimic the development of PD in humans.
-
A therapeutic time window study and the use of ageing animals is necessary to address acute and long-term term effects of CBD in 6-OHDA lesioned rats.
Introduction
Parkinson’s disease (PD) is a neurodegenerative condition that is associated with motor and non-motor symptoms that decrease functioning and quality of life, resulting in high rates of disability and intensive care requirements (WHO, 2022). The main non-motor symptoms of PD include sleep disorders, autonomic dysfunction, fatigue, pain, cognitive deficits, anxiety, and depression (Kalia and Lang Reference Kalia and Lang2016). Despite their high prevalence, non-motor symptoms of PD are scarcely recognised or reported and thus are insufficiently treated (Poewe et al., Reference Poewe, Seppi, Tanner, Halliday, Brundin, Volkmann, Schrag and Lang2017; Santos Garcia et al., Reference Santos García, Jesús, Aguilar, Planellas, García Caldentey, Caballol, Legarda, Hernández Vara, Cabo, López Manzanares, González Aramburu, Ávila Rivera, Catalán, López Díaz, Puente, García Moreno, Borrué, Solano Vila, Álvarez Sauco, Vela, Escalante, Cubo, Carrillo Padilla, Martínez Castrillo, Sánchez Alonso, Alonso Losada, López Ariztegui, Gastón, Kulisevsky, Menéndez González, Seijo, Rúiz Martínez, Valero, Kurtis, de Fábregues-Boixar, González Ardura, Prieto Jurczynska, Martinez-Martin and Mir2019). Anxiety is present in approximately 40-60% of PD patients and appears to be commonly associated with depression (Martínez-Martín and Damián, Reference Martínez-Martín and Damián2010; Schapira et al., Reference Schapira, Chaudhuri and Jenner2017). Depression, apathy, and anhedonia occur in approximately 35% of PD patients (Aarsland et al., Reference Aarsland, Påhlhagen, Ballard, Ehrt and Svenningsson2011). Cognitive impairments are seen as a sign of PD progression and are present in 25-30% of patients (Schapira et al., Reference Schapira, Chaudhuri and Jenner2017; Hayes et al., Reference Hayes, Fung, Kimber and O’Sullivan2019). Notably, dementia (Camicioli et al., Reference Camicioli, Moore, Kinney, Corbridge, Glassberg and Kaye2003) and depressive symptoms have been linked to a reduction of neurogenesis in patients with PD (Lim et al., Reference Lim, Bang and Choi2018).
The pharmacological treatment of PD involves dopamine precursors (e.g. levodopa) and dopamine degradation inhibitors (e.g. monoaminoxidase inhibitors; Alborghetti and Nicoletti, Reference Alborghetti and Nicoletti2019). Although these drugs can attenuate many symptoms of PD, their efficacy gradually decreases over time, accompanied by potential side effects (Cilia et al., Reference Cilia, Cereda, Klersy, Canesi, Zecchinelli, Mariani, Tesei, Sacilotto, Meucci, Zini, Ruffmann, Isaias, Goldwurm and Pezzoli2015). Moreover, non-motor manifestations (e.g. psychosis) can be worsened by dopaminergic medications. Conventional antidepressants do not always show satisfactory clinical effectiveness in PD (Lee and Gilbert, Reference Lee and Gilbert2016). Therefore, the identification of new therapeutic strategies that are able to counteract motor and non-motor symptoms of PD is a major challenge in the field.
Cannabidiol (CBD), one of over 100 phytocannabinoids that have been identified in Cannabis sativa, has received much research attention in recent years because of its multimodal pharmacological profile and remarkable safety profile (Crippa et al., Reference Crippa, Guimarães, Campos and Zuardi2018). Several studies have reported neuroprotective actions of CBD in Alzheimer’s disease (Watt and Karls, Reference Watt and Karl2017), cerebral ischaemia (Mishima et al., Reference Mishima, Hayakawa, Abe, Ikeda, Egashira, Iwasaki and Fujiwara2005; Hayakawa et al., Reference Hayakawa, Mishima, Irie, Hazekawa, Mishima, Fujioka, Orito, Egashira, Katsurabayashi, Takasaki, Iwasaki and Fujiwara2008; Meyer et al., Reference Meyer, Bonato, Mori, Mattos, Guimarães, Milani, de Campos and de Oliveira2021; Khaksar et al., Reference Khaksar, Bigdeli, Samiee and Shirazi-Zand2022), traumatic brain injury (Singh and Neary, Reference Singh and Neary2020), and spinal cord injury (Perez et al., Reference Perez, Cartarozzi, Chiarotto, Guimarães and Oliveira2021). In Parkinsonian patients with no psychiatric comorbidities, CBD improved motor and general symptoms and ameliorated quality of life and well-being (Chagas et al., Reference Chagas, Zuardi, Tumas, Pena-Pereira, Sobreira, Bergamaschi, dos Santos, Teixeira, Hallak and Crippa2014). Experimentally, CBD significantly reduced dopaminergic cell death in rats that were injected with 6-hydroxydopamine (6-OHDA) in the medial forebrain bundle (MFB; Lastres-Becker et al., Reference Lastres-Becker, Molina-Holgado, Ramos, Mechoulam and Fernández-Ruiz2005). CBD also reduced α-synuclein accumulation and restored 6-OHDA depleted dopaminergic neurons in the transgenic C. elegans model of PD (Muhammad et al., Reference Muhammad, Liu, Wang, Zhao, Zhou, Yang and Li2022). When administered locally in the masseter in rats, CBD attenuated the enhancement of nociception in animals with selective loss of the dopaminergic nigrostriatal pathway that was induced by 6-OHDA lesions (Vivanco-Estela et al., Reference Vivanco-Estela, Dos-Santos-Pereira, Guimaraes, Del-Bel and Nascimento2021). An intrapallidal injection of CBD reduced motor asymmetry in 6-OHDA hemiparkinsonian rats and improved fine motor skills in the contralateral limb (Patricio et al., Reference Patricio, Morales Dávila, Patricio-Martínez, Arana Del Carmen, Martínez, Aguilera, Perez-Aguilar and Limón2022). However, whether CBD produces neuroprotective effects on non-motor symptoms of 6-OHDA lesions remains to be determined.
This study aimed to investigate the effect of CBD on non-motor symptoms of rats lesioned with 6-OHDA into the SNpc. Dopaminergic neuron loss was quantified by determining tyrosine hydroxylase (TH) immunoreactivity in the SNpc, ventral tegmental area (VTA), and striatum. Behavioural dysfunction was estimated using a battery of tests, including the open field test (OFT), object recognition test (ORT), novelty-suppressed feeding test (NSF), and forced swim test (FST). To investigate dopaminergic lesions that influence hippocampal neurogenesis, the expression of newborn hippocampal neurons was evaluated by the immunohistochemical detection of doublecortin (DCX)-immunoreactive (IR) neurons. Neuroinflammation was evaluated by Iba-1 (microglia marker) and GFAP (astrocyte marker) expression in the striatum and hippocampus.
Methods
Animals
Male 3-month-old Wistar rats (280-320 g) were obtained from the central vivarium of the State University of Maringá. The animals were acclimated to a controlled temperature (22°C ± 1°C) and a 12 h/12 h light/dark cycle (lights on at 7:00 AM) for 2 weeks before the experiments. The animals were housed in groups (n = 5–10/group) in plastic cages and given standard commercial chow (Nuvilab, Quimtia, PR, Brazil) and tap water ad libitum. The local Ethics Committee on Animal Experimentation of the State University of Maringá approved (CEUA no. 8156290119) the experimental procedures according to the guidelines of the U.S. National Institutes of Health and Brazilian College for Animal Experimentation. All efforts were made to minimise the number of animals used and their suffering.
Drugs
CBD (THC Pharma, Frankfurt, Germany) was dissolved in 2% Tween 80 (Synth, Maringá, Brazil) in sterile isotonic saline (vehicle). Desipramine and 6-OHDA were obtained from Sigma-Aldrich (Sigma, St Louis, MO, USA). Desipramine was dissolved in sterile saline. 6-OHDA was dissolved in a vehicle (sterile saline with 0.2% ascorbic acid). 6-OHDA was prepared according to established doses that were shown to promote significant dopaminergic neuron loss in the SNpc (Bonato et al., Reference Bonato, Bassani, Milani, Vital and de Oliveira2018).
Stereotaxic surgery
Thirty minutes before stereotaxic surgery, the animals received an intraperitoneal (i.p.) injection of desipramine (25 mg/kg) to protect noradrenergic neurons (Padovan-Neto et al., Reference Padovan-Neto, Echeverry, Tumas and Del-Bel2009). The animals were then anaesthetised with Equitesin (3 ml/kg, i.p.; 1% sodium thiopental, 4.25% chloral hydrate, 2.13% magnesium sulphate, 42.8% propylene glycol, and 3.7% ethanol in water; Vieira et al., Reference Vieira, Bassani, Santiago, de O. Guaita, Zanoveli, da Cunha and Vital2019), and the skulls were fixed in a stereotaxic frame (Kopf 957, Kopf Instruments, Tujunga, CA, USA). Bilateral infusions of 6-OHDA were made in a single dose directly in the SNpc (4 μg/μl) using a 27-gauge stainless-steel needle that was connected to a 5 μl Hamilton syringe (Hamilton Company, Reno, NV, USA) according to the following coordinates: SNpc (anterior/posterior, −5.0 mm from bregma; medial/lateral, ± 2.1 mm from midline; dorsal/ventral, −8.0 mm from the skull) (Paxinos and Watson, Reference Paxinos and Watson2007). The flow of the infusions was controlled by an electronic pump (Insight, Ribeirão Preto, Brazil) at a rate of 0.25 μl/min over 4 min (Bonato et al., Reference Bonato, Bassani, Milani, Vital and de Oliveira2018). The injection needle was left in place for an additional 2 min to avoid reflux. After surgeries, the surgical wound was cleaned with chlorhexidine (Sigma-Aldrich, St Louis, MO, USA), and the animals were individually placed in clean cages on warming pads until recovery. No analgesic or anaesthetic antagonists were administered because these treatments could interfere with lesion development and thus impact functional outcomes. After recovery, the rats were returned to group housing (Padovan-Neto et al., Reference Padovan-Neto, Echeverry, Tumas and Del-Bel2009). During the 7 days following surgeries, the animals had free access to laboratory rat chow that was moistened with water to make a paste. The rat chow and water were placed inside the cages to make them easily available and allow the animals to recover. Further, the animals received i.p. vehicle or CBD (10 or 30 mg/kg injections, once a day for 13 days).
Experimental design
The animals were randomly distributed into 4 experimental groups: Saline + Vehicle (Saline + Veh or Sham grou), 6-OHDA + Veh, 6-OHDA + CBD10, and 6-OHDA + CBD30. The animals received intraperitoneal (i.p.) vehicle or CBD (10 or 30 mg/kg injections for 13 days). The CBD doses were determined by referencing prior studies that demonstrated the neuroprotective effects of CBD following brain injury (Mori et al., Reference Mori, Meyer, Soares, Milani, Guimarães and de Oliveira2017; Meyer et al., Reference Meyer, Bonato, Mori, Mattos, Guimarães, Milani, de Campos and de Oliveira2021). Behavioural testing was conducted from 8:00 AM to 12:00 AM. The OFT, ORT, NSF, and FST were performed on days 14, 15, 19, and 22 after the 6-OHDA intra-nigral infusion, respectively (Fig. 1). The time points used for behavioural characterisation after SNpc lesion were based in previous studies (Santiago et al., Reference Santiago, Barbieiro, Lima, Dombrowski, Andreatini and Vital2010; 2014; Bonato et al., Reference Bonato, Bassani, Milani, Vital and de Oliveira2018). Animals’ behaviours were monitored and recorded using a video camera that was positioned above the apparatuses. The images were later analysed using the ANYmaze contrast-sensitive video monitoring system (Stoelting, Wood Dale, IL, USA). After behavioural testing, the animals’ brains were removed and processed for histological analyses.

Figure 1. Experimental design. Vehicle or 6-OHDA was bilaterally injected in the substantia nigra pars compacta (SNpc) according to coordinates from the Paxinos and Watson atlas (2007). The animals received intraperitoneal cannabidiol (CBD) 10 or 30 mg/kg injections for 13 days and were sequentially evaluated in the open field (OF) test, object recognition test (ORT), novel suppressing feeding test (NSF) and forced swim test (FST). The behavioural testing was conducted from day 14 to day 22 after the surgeries. The animals were euthanised on day 22, and brains were removed for immunohistochemistry assays.
Behavioural testing
Open field test. The OFT evaluates locomotion and exploratory behaviour. The OF consisted of a circular arena with an 80 cm diameter and 40 cm high wall. On day 14 after the lesion, each rat was gently moved from its home cage and immediately placed in the centre of the OF. The rat was allowed to freely explore the apparatus for 5 min. The distance travelled (in metres), number of entries, and time spent in the centre of the arena were recorded.
Object recognition test. The ORT is based on the rodent’s instinctive preference for novelty, thus allowing assessments of an animal’s recognition memory (Akkerman et al., Reference Akkerman, Blokland, Reneerkens, van Goethem, Bollen, Gijselaers, Lieben, Steinbusch and Prickaerts2012). On the first day, the animals were placed on a round platform (80 cm diameter, 40 cm high wall) for 5 min for habituation. After two identical objects were placed symmetrically on the platform, the animal was given another 5 min for free exploration. Twenty-four hours later, the animals were returned to the platform with the objects from the previous day, and they were allowed to explore them for 4 min. After a 1 h interval, the animals were returned to the platform, but one of the objects was replaced with a new object, which started the test session. Each animal was given 4 min to explore the new and old (familiar) objects. The exploration time for each object was recorded. Exploration was considered when the animal was at least 2 cm from the object or touched it. Animals that had an exploration time of fewer than 5 s were discarded from the test because this memory assessment requires sufficient exploratory behaviour for reliable performance (Akkerman et al., Reference Akkerman, Blokland, Reneerkens, van Goethem, Bollen, Gijselaers, Lieben, Steinbusch and Prickaerts2012). The following parameters were evaluated: time spent by each animal exploring the familiar object and time spent exploring the new object. The exploration index (D2) was calculated using the following formula: (new object exploration time – familiar object exploration time) / (new object exploration time + familiar object exploration time).
Novel suppressed feeding test. The NSF has been used to assess anxiety-like and depressive-like behaviours (Blasco-Serra et al., Reference Blasco-Serra, González-Soler, Cervera-Ferri, Teruel-Martí and Valverde-Navarro2017). This test introduces an additional element of motivation, in which the animal is deprived of food for a long period. Twenty-four hours before the test, the animal’s food was removed from the cage. The next day, the test was performed on a square platform (70 cm × 70 cm) on which each animal was placed individually. The room lights were turned off, and a spotlight was directed only towards the centre of the platform on a food pellet. The animal was allowed to remain on the platform for 10 min or until the moment when it picked up the pellet. The animal was then transferred to a home cage with previously weighed food pellets and allowed to feed for 10 min. After this period, the food was removed and weighed again to record consumption. After the end of the test, the animals were returned to their cages with food and water available ad libitum. The following parameters were analysed: latency (time for the animal to pick up the food) and consumption in the home cage (weight of the food before feeding – the weight of the food after feeding). The parameters were quantified manually.
Forced swim test. The FST identifies passive coping strategies in rodents (Dalla et al., Reference Dalla, Pitychoutis, Kokras and Papadopoulou-Daifoti2011). The test was conducted in two sessions. In the training session, the rats were placed in a tank (25 cm diameter, 65 cm height) that was filled with water at a temperature of 24°C ± 1°C and depth of 30 cm for 15 min. Twenty-four hours after the training session, the rats were subjected to the test session for 5 min, which was videotaped for subsequent quantification of the latency and immobility time (in seconds; i.e. the absence of motion of the entire body and only small movements necessary to keep the animal’s head above the water). The water was changed after each animal to avoid the influence of urinary or faecal material.
Immunohistochemistry
Five to eight animals were randomly chosen from each experimental group, and their brains were processed for immunohistochemistry. Briefly, the animals were deeply anaesthetised with sodium thiopental (50 mg/kg; Thiopentax, Cristália, SP, Brazil) and then transcardially perfused with phosphate-buffered saline (PBS) followed by 4% paraformaldehyde in 0.2 M phosphate buffer. The brains were removed, postfixed in the same fixative for 2 h, and cryoprotected by immersion in 30% sucrose for 48 h before snap freezing. Frozen tissue was serially sectioned on a cryostat (Cryocut 1800, Reichert-Jung, Heidelberg, Germany) into 30 μm coronal sections that were collected in replicates in Eppendorf tubes that contained 0.01 M PBS with 0.01% sodium azide. The brain sections were quenched in 1% H2O2 for 30 min and then blocked with 2% bovine serum albumin (BSA) in PBS for 60 min. The sections were incubated with mouse polyclonal anti-TH antibody (1 : 2,000, catalogue no. AB152, Merck Millipore, Darmstadt, Germany), goat polyclonal anti-DCX antibody (1 : 1,000, catalogue no. sc-8066, Santa Cruz Biotechnology, Santa Cruz, CA, USA), rabbit polyclonal anti-Iba-1 antibody (1 : 500, catalogue no. 0019-19741, Wako Osaka, Japan) or rabbit anti-GFAP (1:500, catalogue no. sc-2042, Santa Cruz Biotechnology, Santa Cruz, CA, USA) in PBS that contained 0.3% Triton X-100 for 48 h at 4°C. The sections were then incubated with respective biotinylated secondary antibodies (1:500, anti-mouse [catalogue no. sc2039], anti-goat [catalogue no. sc-2042] and anti-rabbit [catalogue no. 2492] Santa Cruz Biotechnology, Santa Cruz, CA, USA), for 2 h. The signals were visualised using avidin-biotin complex (Vector Laboratories, Burlingame, CA, USA), 3,3’-diaminobenzidine (DAB) as the chromogen, and 0.05% H2O2. For the Iba-1 and GFAP assays, 1% Ni2SO4 was added to the revelation solution. The sections were then mounted on gelatin-coated slides, dehydrated in ethanol, and coverslipped with Permount mounting medium.
For semi-quantitative analysis, the slides were previously coded and evaluated by an experimenter who was blind to treatments. The slides were analysed with an optical microscope (Olympus, BX41, PA, USA) that equipped with an Olympus QColor camera. Immunoreactive cells were quantified by capturing photomicrographs from the the SNpc, VTA, hippocampus, and striatum. ImageJ software (National Institutes of Health, Bethesda, MD, USA) was utilised to count the number of immunoreactive cells or the integrated optical density (IOD), in pre-fixed areas. For IOD measurements, after acquiring the images, they were converted to a 16-bit greyscale image, the background was subtracted and the threshold for positive signals was determined. The two cerebral hemispheres were evaluated, and the average between them was obtained. The analysis was conducted with four to six sections per animal.
Doublecortin-IR cells were counted throughout the SGZ of the dentate gyrus of the hippocampus (bregma −3.14 to −4.52 mm; Paxinos and Watson, Reference Paxinos and Watson2007). For each section, the DG area was obtained, and the number of DCX-IR cells was counted. To estimate the total number of immunoreactive cells in the SGZ, a modified unbiased stereological procedure was used where the total number of positive cells counted per DG is multiplied by the reciprocal of the sampling factor (Bonato et al., Reference Bonato, Bassani, Milani, Vital and de Oliveira2018). Therefore, DCX-IR cells were counted on every 6th section of the hippocampus and the number of counted cells was multiplied by 6 to obtain an estimate of the total number of positive cells per SGZ. The SGZ of the DG was defined as the region between the hilus and the granular cell layer (GCL): two GCL cell widths into the hilus and the inner half of the GCL (Lau et al., Reference Lau, Hebert, Vani, Walling, Hayley, Lagace and Blundell2016).
Doublecortin-IR neurons were classified into six different categories according to their dendrite morphology (Pl ümpe et al., Reference Plümpe, Ehninger, Steiner, Klempin, Jessberger, Brandt, Römer, Rodriguez, Kronenberg and Kempermann2006): A (neuron with no processes), B (neuron with very short processes), C and D (neurons with intermediate processes, with D being more developed than C), and E and F (which corresponded to more mature neurons; E neurons had apparent branches in the molecular layer of the SGZ, and F neurons had several branches along the dendritic tree). The results are presented as the sum of A + B (proliferative stage), C + D (intermediate stage), and E + F (post-mitotic stage; Fig. 6A).
Statistical analysis
Statistica 8.0 software (StatSoft, Palo Alto, CA, USA) was used for the statistical analysis. A mortality curve was generated to analyse survival data and the Long-rank test (χ2) was used to assess differences in animal survival. First, the behavioural and histological data were examined to verify normality (Shapiro-Wilk test) and homoscedasticity (F test to compare variances). The behavioural data were analysed using one-way analysis of variance (ANOVA), followed by the Tukey’s multiple-comparison post hoc test. In the ORT, functional memory within groups (i.e. a D2 value that differed significantly from zero) was analysed with a two-tailed one sample t-test (Soares et al., Reference Soares, De Vry, Steinbusch, Milani, Prickaerts and Weffort de Oliveira2016). The data are expressed as mean ± SEM. Values of p < 0.05 were considered statistically significant.
Result
Mortality rate
In total, 71 animals were used: Saline + Veh (n = 11), 6-OHDA + Veh (n = 25), 6-OHDA + CBD10 (n = 22), and 6-OHDA + CBD30 (n = 13). As shown in Fig. 2, the mortality rate was: Saline + Veh 15.5 % (1 of 11 animals), 6-OHDA + Veh 52.0 % (13 of 25 animals), 6-OHDA + CBD10 50% (11 of 22 animals) and 6-OHDA + CBD30 38.46 % (5 of 13 animals). 6-OHDA + Veh (χ2 = 4.86, p = 0.027) and 6-OHDA + CBD10 (χ2 = 4.66, p = 0.03) groups were different from Saline + Veh group. CBD 30 mg/kg did not protect the animals from 6-OHDA-induced death since there was no significant difference between 6-OHDA + Veh and 6-OHDA + CBD30 groups (χ2 = 0.43, p = 0.51).
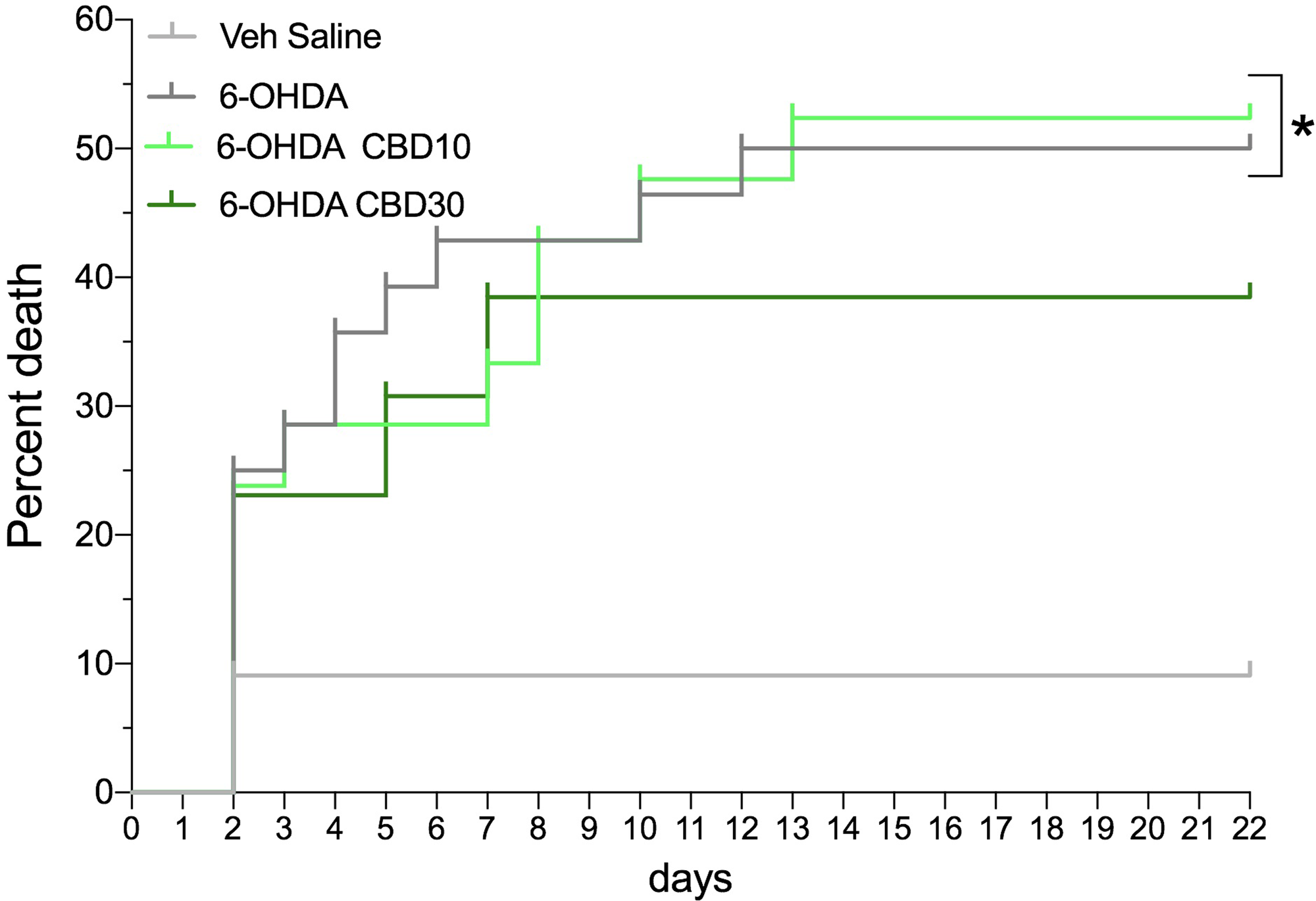
Figure 2. Mortality curve. Mortality rate expressed as a percentage over time (days) of the experimental groups. *p < 0.05 compared with saline + vehicle group (Long-rank test).
CBD protects the SNpc dopaminergic neurons from bilateral 6-OHDA infusion into the SNpc
Dopaminergic neuronal loss was evaluated in the SNpc, striatum, and VTA 22 days after bilateral 6-OHDA infusion into the SNpc (Fig. 3). Animals that did not present bilateral lesions were removed from the analysis. ANOVA revealed significant differences among the experimental groups concerning TH-IR cells into SNpc (F3,35 = 12.60, p < 0.0001), striatum (F3,35 = 2.54, p = 0.007), and VTA (F3,35 = 4.0, p = 0.02). There was a decrease in the TH-IR in the SNpc, striatum, and, VTA in 6-OHDA + Veh group when compared to Veh + saline group (p < 0.05). CBD 10 mg/kg did not protect dopaminergic neurodegeneration in the SNpc, VTA or striatum (p > 0.05). In contrast, CBD 30 mg/Kg prevented dopaminergic neuronal loss in the SNpc (p = 0.03) and attenuated dopaminergic neuronal loss in the striatum and VTA, albeit this effect did not reach the 5% significance level.
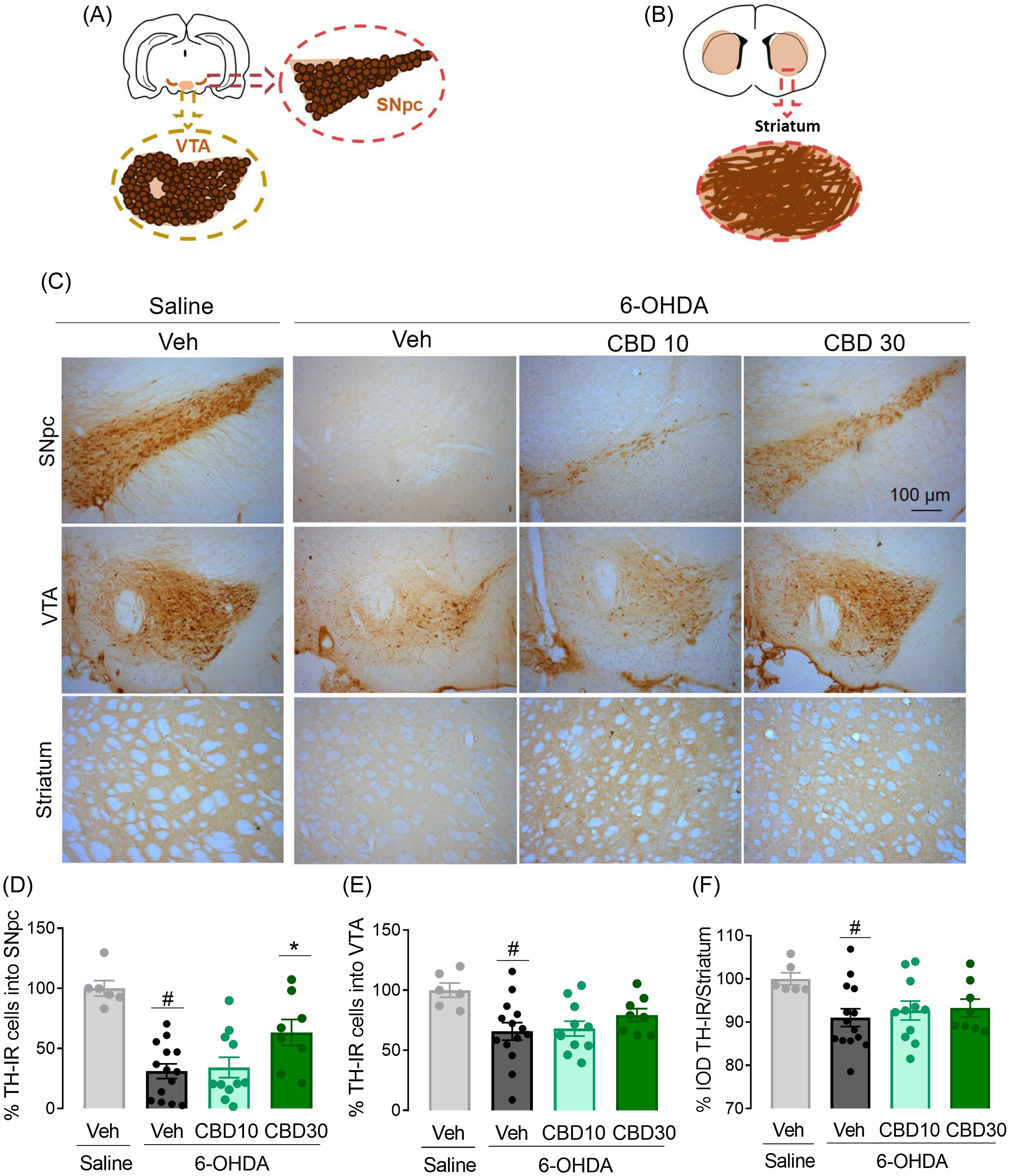
Figure 3. CBD protects the SNpc dopaminergic neurons from bilateral 6-OHDA infusion into the SNpc. Representative diagrams of tyrosine-hydroxylase-immunioreactivity (TH-IR) cells in the substantia nigra pars compacta (SNpc) and ventral tegmental area (VTA) (A), and striatum (B) according to the Paxinos and Watson atlas (2007). Representative photomicrographs of TH-IR cells in the SNpc, VTA and striatum 22 days after 6-OHDA injection (C). The number of TH-IR cells in the SNpc (D), VTA (E) and striatum (F) are represented as % of the controls. **p < 0.001 when compared to saline + veh group and #p < 0.05 when compared to 6-OHDA + veh group (ANOVA followed by the Tukey’s post hoc test).
Effects of CBD on non-motor symptoms following bilateral 6-OHDA infusion into the SNpc
The OF has been used to evaluate the locomotor activity of the animals as well as anxiety-related behaviours. As shown in Fig. 4A, ANOVA detected a significant difference among the experimental groups concerning the distance travelled in the OF (F 3,36 = 3.75, p = 0.02). The 6-OHDA + Veh group presented a decrease in the distance travelled when compared to Saline + Veh group (p, 0.05). No other significant change was observed concerning the number of entries (F 3,36 = 1.68, p = 0.19) and time (F3,36 = 1.09, p = 0.37) in the OF centre between sham (Saline + Vehicle) and lesioned animals.
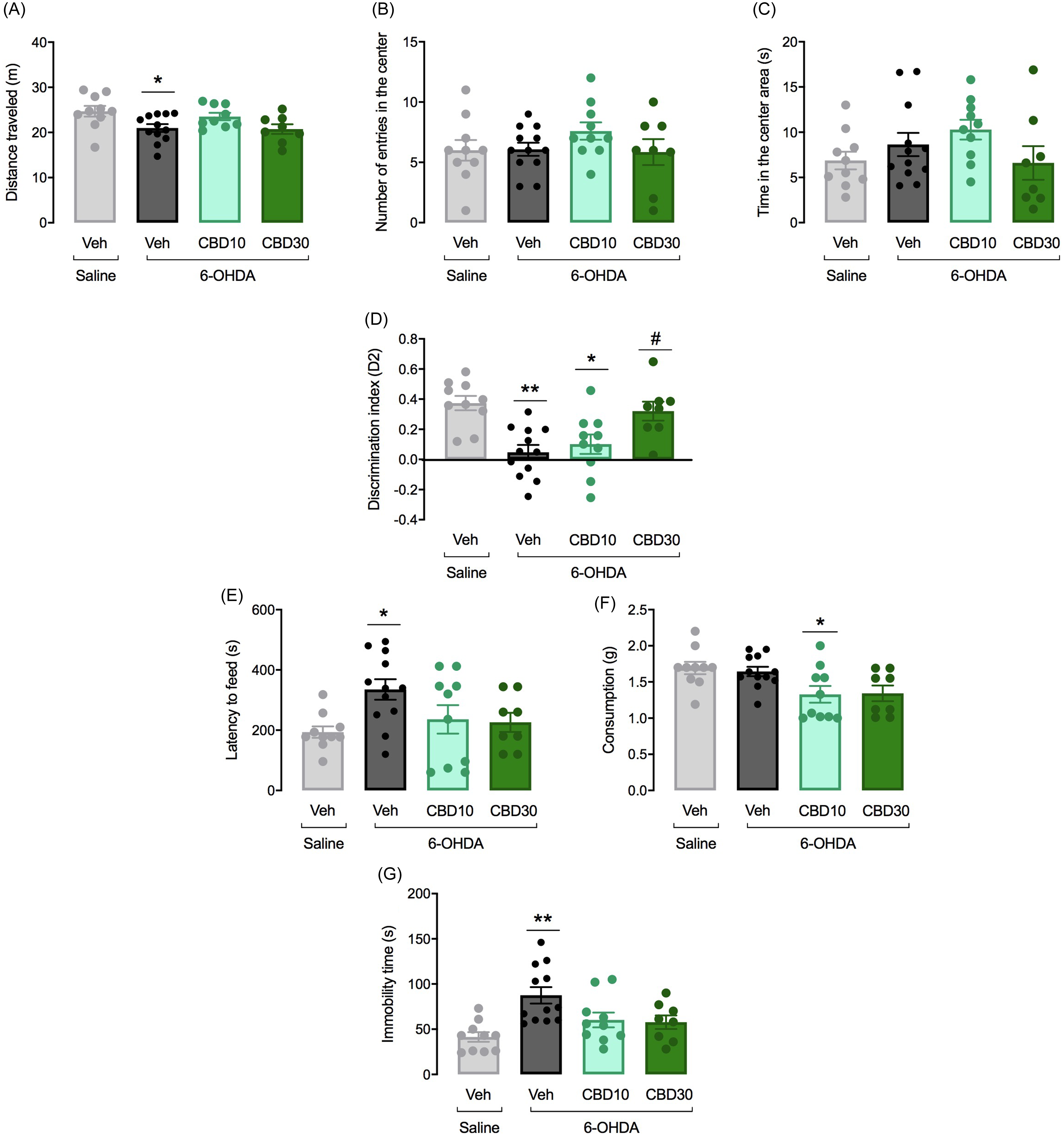
Figure 4. Effects of CBD on non-motor symptoms following bilateral 6-OHDA infusion into the SNpc. Motor activity was evaluated in the open field (OF) by measuring the distance travelled in metres (A). Anxiety-like behaviour was evaluated by measuring the number of entries (B) and the time (sec) (C) spent in the centre of the OF. Hippocampus-dependent memory was analysed in the object location test (ORT) using the D2 exploration index (new object exploration time – familiar object exploration time) / (new object exploration time + familiar object exploration time) (D). The latency to feed (E) and food consumption (F) were registered in the NSF test. Despair-like behaviours were detected in the forced swim test (FST) by measuring the immobility time (G). The bars represent the mean ± SEM in the different groups (n = 10-12/group). **p < 0.001 and *p < 0.05 different from the saline + vehicle group. #p < 0.05 different from 6-OHDA + veh group (one-way ANOVA followed by the Tukey’s post hoc test).
Fig. 4D shows the effects of 6-OHDA on memory performance in the ORT. To investigate which groups had functional recognition memory, the D2 value in the ORT was compared to 0. Only the control (t = 7. 811, df = 18, p < 0.0001) and the 6-OHDA + CBD30 (t = 5.708, df = 16, p < 0. 0001) groups were different from zero. During the test session, the 6-OHDA + Veh group explored less the new object compared with the Saline + Veh group (t = 4.72, df = 20, p < 0.001), indicating that lesioned rats did not retain recognition memory of the familiar object. ANOVA showed significant differences in the discrination index among the groups (F 3,36 = 8.53, p < 0.001). 6-OHDA lesioned, 6-OHDA + CBD10 and 6-OHDA + CBD30 groups presented a decrease in the discrimination index when compared to controls (p < 0.05). CBD 30 mg/kg prevented the memory deficits induced by SN 6-OHDA lesion (p = 0.009) when compared to 6-OHDA + Veh group.
In the NSF test, the 6-OHDA lesion induced changes in the latency to feed when compared to sham animals (F3,36 = 3.37, p = 0.03, Fig. 4E). The 6-OHDA + veh group showed an increase latency to feed when compared to the control group (p = 0.03). Also for the food consumption, there was a significant difference among the groups (F3,36 = 4.33, p = 0.01, Fig. 4E). While 6-OHDA infusion did not affect the animal’s food consumption (p > 0.05) the 6-OHDA group that received CBD10 mg/Kg presented a decrease consumptiom (p = 0.04) in comparison to Saline + veh group.
The immobility time in the FST reflects despair-like behaviours. ANOVA revealed significant differences among groups (F 3,36 = 6.48, p = 0.001). As seen in Fig. 4G, bilateral 6-OHDA infusion into the SNpc results in an increased immobility time of lesioned rats when compared to controls (p < 0.001). Although CBD 10 and 30 mg/Kg attenuated the effects of 6-OHDA lesion, this effect did not reach statistical significance.
CBD reduces neuroinflammation induced by bilateral 6-OHDA infusion into the SNpc
Neuroinflammation was assessed by analysing the expression of Iba-1 (microglia) and GFAP (astrocytes) in the striatum and CA1 and CA3 hippocampal subfields (Fig. 5). Analysis of Iba-1-IR cells revealed significant differences in the total number of microglial cells in the striatum (F 3,16 = 3.82, p = 0.03) and CA1 (F 3,16 = 6.4, p = 0.005) of lesioned rats, while no significant variance was observed in the CA3 hippocampal subfield (F3,16 = 0.94, p = 0.44). Additionally, ANOVA demonstrated notable differences in GFAP-IR in the CA1 region of the hippocampus (F 3,16 = 4.1, p = 0.02), with no significant changes noted in the CA3 hippocampal subfield (F 3,16 = 0.94, p = 0.44) or the striatum (F 3,16 = 3.16, p = 0.63) of rats infused with intra-nigral 6-OHDA.

Fig. 5. CBD decreases neuroinflammation induced by bilateral 6-OHDA infusion nto the SNpc. Representative photomicrographies of Iba-1 and GFAP immunohistochemistry in coronal brain sections of the striatum (A) and CA1 hippocampal subfield (B) of rats. Number of Iba-1-IR cells in the striatum (C) and CA1 hippocampal subfield (E). % of control of IOD GFAP-IR in the striatum (D) and CA1 hippocampal subfield (F) of rats. The bars represent the mean ± SEM of the experimental groups. *p < 0.05 different from the saline + vehicle group. #p < 0.05 different from 6-OHDA + veh group (one-way ANOVA followed by by the Tukey’s post hoc test).
CBD favours newborn neurons maturation in the hippocampus of 6-OHDA lesioned rats
Fig. 6 shows the DCX-IR cells in ZSG of DG, 22 days after bilateral 6-OHDA intra-nigral infusion. The number of DCX-IR neurons was not different among the experimental groups (F 3,20 = 1.64, p = 0.21). However, ANOVA revealed significant differences in DCX-IR cell dendrite classification among the experimental groups, in the stages A + B (F 3,20 = 4.19, p < 0.05), C + D (F 3,20 = 10.58, p < 0.001) and E + F (F 3,20 = 23.56, p < 0.001). The treatment with CBD 10 mg/kg increased the number of cells in the A + B (p < 0.05) and C + D (p < 0.001) stages (Fig. 5E and 5F). In the post-mitotic stage (E + F), the groups 6-OHDA + Veh and 6-OHDA + CBD10 presented a decreased number of DCX-IR cells compared with the Saline + Veh group (p < 0.001; Fig. 6G). In contrast, the treatment with CBD 30 mg/kg increased the number of DCX-IR cells in the postmitotic stage when compared with 6-OHDA + Veh group (p < 0.001).
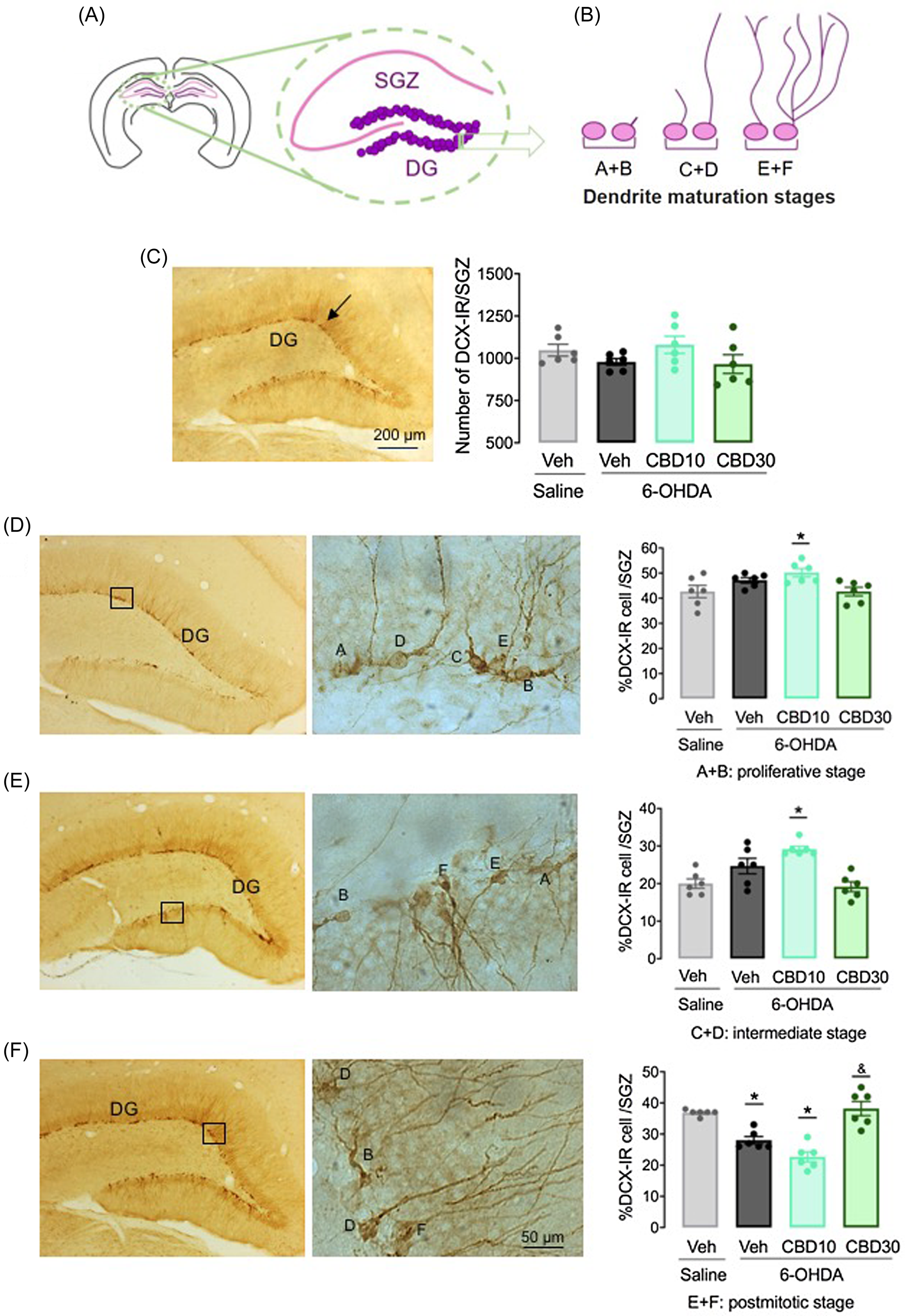
Figure 6. CBD favours newborn neurons maturation in the hippocampus of 6-OHDA lesioned rats. Representative diagrams of coronal brain sections of the hippocampus (A) and classification of DCX-IR neuron dendrite morphology (B). Number of DCX-IR cells in the subgranular zone of the hippocampus (D) and percentage of DCX-IR cells per stage, that is, proliferative stage (E), intermediate stage (F) and postmitotic stage (G). The bars represent the mean ± SEM of the experimental groups. **p < 0.001 and *p < 0.05 different from the saline + vehicle group. ##p < 0.05 different from 6-OHDA + veh group (one-way ANOVA followed by by the Tukey’s post hoc test).
Discussion
The present study investigated neuroprotective effects of CBD in rats that received bilateral intranigral 6-OHDA lesions. 6-OHDA-lesioned rats exhibited memory impairments in the ORT and depressive-like behaviour in the NSF and FST paradigms. In parallel, the animals exhibited dopaminergic neuronal loss in the SNpc, striatum, and VTA. CBD decreased the mortality rate, prevented memory impairments, and attenuated despair-like behaviours in 6-OHDA-lesioned animals. Moreover, CBD decreased dopaminergic neuronal loss in the SNpc, attenuated neuroinflammation and favoured newborn neuron maturation in the hippocampus in Parkinsonian rats.
Toxin administration in specific brain regions in rodents, including the SNpc, MFB, and striatum, has been widely reported to cause the degeneration of dopaminergic neurons and mimic some clinical aspects of PD (Simola et al., Reference Simola, Morelli and Carta2007; Chia et al., Reference Chia, Tan and Chao2020). Specifically, bilateral infusions of 6-OHDA in the SNPc have been shown to produce dopaminergic neurodegeneration and consistent depressive-like behaviours in rats (Santiago et al., Reference Santiago, Barbieiro, Lima, Dombrowski, Andreatini and Vital2010; Bonato et al., Reference Bonato, Bassani, Milani, Vital and de Oliveira2018). One drawback of this lesion model, however, is the high mortality rate after bilateral intranigral 6-OHDA infusions. In the present study, bilateral intranigral 6-OHDA infusions resulted in a 52% mortality rate. CBD (30 mg/kg) decreased the mortality rate in 6-OHDA-lesioned rats. This finding is consistent with previous studies. In an in vivo model of generalised seizures that were induced by pentylenetetrazol, CBD decreased seizures and mortality compared with vehicle-treated animals (Jones et al., Reference Jones, Hill, Smith, Bevan, Williams, Whalley and Stephens2010). CBD also improved outcomes and prevented mortality in a severe seizure model of soman exposure in female mice (Kundrick et al., Reference Kundrick, Marrero-Rosado, de Araujo Furtado, Stone, Schultz and Lumley2021). Patra et al. (Reference Patra, Serafeimidou-Pouliou, Bazelot, Whalley, Williams and McNeish2020) recently reported that CBD prevented premature mortality and improved cognition and social interaction in the Scn1a ± mouse model of Dravet syndrome. Furthermore, CBD increased the mean survival time in aluminium phosphide-poisoned rats (Hooshangi Shayesteh et al., Reference Hooshangi Shayesteh, Haghi-Aminjan, Baeeri, Rahimifard, Hassani, Gholami, Momtaz, Salami, Armandeh, Bameri, Samadi, Mousavi, Ostad and Abdollahi2022) and reduced cocaine lethality in mice (Vilela et al., Reference Vilela, Gomides, David, Antunes, Diniz, Moreira Fício de and Menezes2015). Altogether, these findings indicate that CBD might exert a neuroprotective effect by decreasing mortality in rats under different experimental conditions.
Bilateral intranigral 6-OHDA infusions induced dopaminergic neurodegeneration in the SNpc, striatum, and VTA. Previous studies (Lindner et al., Reference Lindner, Cain, Plone, Frydel, Blaney, Emerich and Hoane1999; Blandini et al., Reference Blandini, Levandis, Bazzini, Nappi and Armentero2007) reported that injections of 6-OHDA in the SNpc caused the retrograde degeneration of these cells. In the present study, CBD attenuated neuronal loss only in the SNpc and not in the VTA or striatum. This lack of statistical significance for the effect of CBD in the VTA and striatum may be due to the high interindividual variability observed in the 6-OHDA + Vehicle group. Results of in vivo studies of effects of CBD on dopaminergic cells in animal models of PD are conflicting. The administration of CBD (5 mg/kg) for 5 weeks did not impact dopaminergic neuronal loss in mice that were lesioned with 1-methyl-4-phenyl-1,2,3,6-tetrahydropyridine (MPTP; Celorrio et al., Reference Celorrio, Rojo-Bustamante, Fernández-Suárez, Sáez, Estella-Hermoso de Mendoza, Müller, Ramírez, Oyarzábal, Franco and Aymerich2017). In other study, the daily administration of CBD (3 mg/kg) for 13 days decreased dopamine levels and TH expression in the striatum in rats that received unilateral injections of 6-OHDA in the MFB (Lastres-Becker et al., Reference Lastres-Becker, Molina-Holgado, Ramos, Mechoulam and Fernández-Ruiz2005). These conflicting results could be attributable to differences in experimental methodologies because the magnitude of dopaminergic lesions depends on the type and dose of toxins, the site of injections, whether the lesions are uni- or bilateral, and the age and species of the animals (Tieu, Reference Tieu2011). Yet, one should consider using different CBD dosages.
The OF was used to evaluate motor functions and anxiety-related behaviour that were induced by bilateral intranigral 6-OHDA infusions. 6-OHDA-lesioned rats exhibited a decrease in the distance travelled in the OF compared with the control group. The SNpc lesion was expected to cause hypolocomotion because the nigrostriatal pathway is related to locomotor function. However, this effect is transient, in which motor activity is recovered by compensatory mechanisms 2–3 weeks after the intranigral 6-OHDA lesion (Bové and Perier, Reference Bové and Perier2012; Santiago et al., Reference Santiago, Tonin, Barbiero, Zaminelli, Boschen, Andreatini, Da Cunha, Lima and Vital2015; Bonato et al., Reference Bonato, Bassani, Milani, Vital and de Oliveira2018). No group differences in anxiety-related parameters were detected in the OF, which is consistent with the finding that hemiparkinsonian rats and controls exhibited similar behaviours in the elevated plus maze test of anxiety (Delaville et al., Reference Delaville, Chetrit, Abdallah, Morin, Cardoit, De Deurwaerdère and Benazzouz2012). However, contradictory findings of intranigral 6-OHDA lesion-induced anxiety-like behaviour in rats have been reported (Chen et al., Reference Chen, Liu, Zhang, Feng, Gui, Ali, Wang, Fan, Hou and Wang2011; Vieira et al., Reference Vieira, Bassani, Santiago, de O. Guaita, Zanoveli, da Cunha and Vital2019).
In the present study, 6-OHDA-lesioned rats exhibited memory deficits in the ORT compared with controls. CBD treatment increased exploration time in Parkinsonian rats in the ORT. In agreement, the administration of CBD improved memory in rats (Meyer et al., Reference Meyer, Bonato, Mori, Mattos, Guimarães, Milani, de Campos and de Oliveira2021) and mice (Mori et al., Reference Mori, Meyer, Soares, Milani, Guimarães and de Oliveira2017) that were subjected to cerebral ischaemia. CBD also improved memory in animal models of Alzheimer’s disease including APPxPS1 (Coles et al., Reference Coles, Watt, Kreilaus and Karl2020; Chesorth et al., Reference Chesworth, Cheng, Staub and Karl2022) and aged TAU58/2 transgenic female mice (Kreilaus et al., Reference Kreilaus, Przybyla, Ittner and Karl2022). Effects of CBD on memory performance have been associated with a decrease in neuroinflammation and an increase in levels of trophic factors and proteins that are involved in hippocampal neurogenesis and synaptic plasticity (Mori et al., Reference Mori, Meyer, Soares, Milani, Guimarães and de Oliveira2017; Meyer et al., Reference Meyer, Bonato, Mori, Mattos, Guimarães, Milani, de Campos and de Oliveira2021).
Astrogliosis and microglial activation contribute to neuroinflammation-induced neuronal death in PD (Lee et al., Reference Lee, Lee, Chang and Lee2019). In particular, microglia activation combined with oxidative stress and apoptosis, contributes to the degeneration of dopaminergic neurons (Pabon et al., Reference Pabon, Bachstetter, Hudson, Gemma and Bickford2011). In this study, CBD attenuated the glial response induced by the infusion of 6-OHDA in the SNpc of rats. Decreases in the expression of Iba-1, a microglial marker, were observed in the hippocampus and striatum of lesioned rats. Besides, CBD treatment improved neuronal maturation, potentially enhancing cognitive function in 6-OHDA lesioned rats. We speculate if these effects of CBD effects could be linked to its anti-inflammatory action. In line, in vitro and in vivo investigations have demonstrated CBD’s neuroprotective impact on several experimental conditions by addressing microglia-mediated neuroinflammation. For example, Wang et al., (2022) have shown that a 14-day regimen of CBD treatment precipitates a conspicuous reduction in both apoptosis and neuroinflammation subsequent to the administration of MPTP in mice. These studies demonstrated that CBD exerts its anti-neuroinflammatory effects on microglial cells by downregulating NF-κB dependent signalling pathways and suppressing NADPH oxidase-mediated ROS expression (Dos-Santos-Pereira et al., Reference Dos-Santos-Pereira, Guimarães, Del-Bel, Raisman-Vozari and Michel2020; Yousaf et al., Reference Yousaf, Chang, Liu, Liu and Zhou2022). Whether CBD impacts the NF-κB pathways expression in 6-OHDA lesioned rats needs further investigation.
Concerning the effects on synaptic plasticity, a pro-neurogenic effect has been attributed to CBD. The treatment with CBD (6 weeks) enhanced adult neurogenesis; however, this effect was not present in mice lacking CB1R suggesting the critical role of these receptors in the CBD-mediated actions on hippocampal neurogenesis (Wolf et al., Reference Wolf, Bick-Sander, Fabel, Leal-Galicia, Tauber, Ramirez-Rodriguez, Müller, Melnik, Waltinger, Ullrich and Kempermann2010). Furthermore, the anxiolytic actions of CBD in mice exposed to chronic unpredictable stress were closely associated with the pro-neurogenic effect of CBD. The authors suggested that this phenomenon depends on the facilitation of the endocannabinoid-mediated signalling and subsequent cannabinoid receptors activation (Campos et al., Reference Campos, Ortega, Palazuelos, Fogaça, Aguiar, Díaz-Alonso, Ortega-Gutiérrez, Vázquez-Villa, Moreira, Guzmán, Galve-Roperh and Guimarães2013). Likewise, repeated administration of CBD at low doses (3 mg/kg, i.p.) increased cell proliferation and neurogenesis in the DG and SVZ (Schiavon et al., Reference Schiavon, Bonato, Milani, Guimarães and Weffort de Oliveira2016) and improved memory in ischaemic mice.
The NSF test has been used as a measure of anxiety- and depressive-like behaviours (Blasco-Serra et al., Reference Blasco-Serra, González-Soler, Cervera-Ferri, Teruel-Martí and Valverde-Navarro2017). Intranigral infusions of 6-OHDA promoted an increase in the latency to feed in the NSF 18 days after the lesion. This finding was accompanied by another feature of behavioural despair, reflected by an increase in immobility time in the FST. CBD attenuated effects of 6-OHDA lesions in both the NSF test and FST, indicating an antidepressant-like effects of this treatment. Indeed, CBD has been shown to promote antidepressant-like effect in several experimental conditions. Although the pharmacological mechanism are not entirely clear, the antidepressant-like effect of CBD has been related to serotonin 5-hydroxytryptamine-1A (5-HT1A) receptor activation (Zanelati et al., Reference Zanelati, Biojone, Moreira, Guimarães and Joca2010; Sales et al., Reference Sales, Crestani, Guimarães and Joca2018).
The degeneration of midbrain dopaminergic neurons has been shown to affect several mesolimbic structures, including the hippocampus (Bonito-Oliva et al., Reference Bonito-Oliva, Masini and Fisone2014; Kim et al., Reference Kim, Weerasinghe-Mudiyanselage, Ang, Lee, Kang, Kim, Kim, Kim, Jung, Shin and Moon2022), which is critically involved in memory and emotional control. Hippocampal dysfunction has been reported in neurotoxic and genetic animals models of PD, including 6-OHDA-lesioned rats (Esmaeili-Mahani et al., Reference Esmaeili-Mahani, Haghparast, Nezhadi, Abbasnejad and Sheibani2021) and α-synuclein transgenic mice (Costa et al., Reference Costa, Sgobio, Siliquini, Tozzi, Tantucci, Ghiglieri, Di Filippo, Pendolino, de Iure, Marti, Morari, Spillantini, Latagliata, Pascucci, Puglisi-Allegra, Gardoni, Di Luca, Picconi and Calabresi2012). The loss of dopaminergic neurons that was induced by intranigral 6-OHDA infusions was paralleled by the area-dependent degeneration of noradrenergic and serotonergic systems (Santiago et al., Reference Santiago, Barbieiro, Lima, Dombrowski, Andreatini and Vital2010; Delaville et al., Reference Delaville, Chetrit, Abdallah, Morin, Cardoit, De Deurwaerdère and Benazzouz2012) and a decline of hippocampal synaptic plasticity (Maxreiter et al., Reference Marxreiter, Regensburger and Winkler2013; Lim et al., Reference Lim, Bang and Choi2018). In the present study, DCX immunoreactivity was used to study plastic changes at the level of the dendrite maturation of newborn hippocampal neurons (Brown et al., Reference Brown, Couillard-Després, Cooper-Kuhn, Winkler, Aigner and Kuhn2003; Plümpe et al., Reference Plümpe, Ehninger, Steiner, Klempin, Jessberger, Brandt, Römer, Rodriguez, Kronenberg and Kempermann2006). Intranigral 6-OHDA lesions decreased the number of DCX-IR neurons in the postmitotic stage, indicating that the lesions impacted neuronal differentiation. This effect was prevented by CBD at the dose of 30 mg/kg, but not 10 mg/kg. One possibility is that CBD facilitated neuronal maturation which could impact the establishment of synaptic connectivity and integration of newborn neurons in the hippocampal circuitry. This effect might be particularly important for hippocampus-dependent functions.
CBD exerts its neuroprotective effects through cannabinoid receptor-dependent and -independent mechanisms. In PD models, CBD has been shown to attenuate neuroinflammation and oxidative stress through cannabinoid receptor-independent actions (Lastres-Becker et al., Reference Lastres-Becker, Molina-Holgado, Ramos, Mechoulam and Fernández-Ruiz2005; Patricio et al., Reference Patricio, Morales-Andrade, Patricio-Martínez and Limón2020). Moreover, CBD is known to stimulate hippocampal neurogenesis and synaptic plasticity (Campos et al., Reference Campos, Fogaça, Sonego and Guimarães2016). However, the pharmacological profile of CBD is complex. It is known to act on different molecular targets (Patricio et al., Reference Patricio, Morales-Andrade, Patricio-Martínez and Limón2020). We recently reported the involvement of cannabinoid CB1 and CB2 receptors, serotonin 5-HT1A receptors, and peroxisome proliferator-activated receptor-γ (PPAR-γ) in cognitive and emotional symptoms in mice that were subjected to cerebral ischaemia (Mori et al., Reference Mori, Meyer, da Silva, Milani, Guimarães and Oliveira2021). Remaining to be determined is whether similar mechanisms are involved in neuroprotective effects of CBD observed in 6-OHDA-lesioned rats.
Availability of data and material
Experimental data will be made available under reasonable request.
Acknowledgements
This work was supported by Conselho Nacional de Desenvolvimento Científico e Tecnológico (CNPq), Universidade Estadual de Maringá, Paraná, Brazil, and Coordenação de Aperfeiçoamento de Pessoal de Nível Superior (CAPES). The authors thank Marcos Alberto Trombelli for his technical support.
Author contribution
BAM and RMWO conceived and designed the experiments and wrote the manuscript. BAM, JMB and MCS performed the experiments. JMB and HM helped with data and statistical analysis. EADB made a critical revision of the manuscript before submission to the Journal. All authors discussed and commented on the manuscript.
Competing interests
There is no conflict of interest concerning the present study.
Author’s declarations and consent to participate and consent for publication
This study was carried out at the State University of Maringá in strict accordance with the Brazilian College of Animal Experimentation (COBEA) recommendations. Animal experiments were approved by the local Ethics Committee on Animal Experimentation of the State University of Maringá (animal licence number: CEUA no. 8156290119). All of the co-authors approved the final version of the manuscript and agreed to submit it to Acta Neuropsychiatrica.