Book contents
- Optical Coherence Tomography in Neurological Diseases
- Optical Coherence Tomography in Neurological Diseases
- Copyright page
- Contents
- Contributors
- Preface
- Chapter 1 Introduction to optical coherence tomography in neurological diseases
- Chapter 2 Basic principles of optical coherence tomography
- Chapter 3 Anatomy of the anterior visual pathway
- Chapter 4 Optical coherence tomography in acute optic neuritis
- Chapter 5 Optical coherence tomography and visual outcomes in acute optic neuritis
- Chapter 6 Optical coherence tomography and low-contrast acuity
- Chapter 7 Optical coherence tomography and electrophysiology of the visual pathway
- Chapter 8 Optical coherence tomography and electrophysiology of the optic nerve head
- Chapter 9 Meta-analysis of optical coherence tomography in multiple sclerosis
- Chapter 10 Optical coherence tomography and brain magnetic resonance imaging in multiple sclerosis
- Chapter 11 Optical coherence tomography in neurodegenerative and other neurologic diseases
- Chapter 12 Optical coherence tomography pathologies to know about in clinical practice
- Chapter 13 Optical coherence tomography and retinal segmentation in neurological diseases
- Chapter 14 Optical coherence tomography and retinal pathology in neurologic diseases
- Chapter 15 Retinal inflammation in multiple sclerosis revealed by optical coherence tomography and ophthalmoscopy
- Chapter 16 Optical coherence tomography and optic nerve magnetic resonance imaging in demyelinating diseases
- Chapter 17 Optical coherence tomography in neurologic clinical trials
- Chapter 18 Optical coherence tomography in a multi-center setting: quality control issues
- Chapter 19 Future technological advances in optical coherence tomography
- Index
- References
Chapter 19 - Future technological advances in optical coherence tomography
Published online by Cambridge University Press: 05 May 2015
- Optical Coherence Tomography in Neurological Diseases
- Optical Coherence Tomography in Neurological Diseases
- Copyright page
- Contents
- Contributors
- Preface
- Chapter 1 Introduction to optical coherence tomography in neurological diseases
- Chapter 2 Basic principles of optical coherence tomography
- Chapter 3 Anatomy of the anterior visual pathway
- Chapter 4 Optical coherence tomography in acute optic neuritis
- Chapter 5 Optical coherence tomography and visual outcomes in acute optic neuritis
- Chapter 6 Optical coherence tomography and low-contrast acuity
- Chapter 7 Optical coherence tomography and electrophysiology of the visual pathway
- Chapter 8 Optical coherence tomography and electrophysiology of the optic nerve head
- Chapter 9 Meta-analysis of optical coherence tomography in multiple sclerosis
- Chapter 10 Optical coherence tomography and brain magnetic resonance imaging in multiple sclerosis
- Chapter 11 Optical coherence tomography in neurodegenerative and other neurologic diseases
- Chapter 12 Optical coherence tomography pathologies to know about in clinical practice
- Chapter 13 Optical coherence tomography and retinal segmentation in neurological diseases
- Chapter 14 Optical coherence tomography and retinal pathology in neurologic diseases
- Chapter 15 Retinal inflammation in multiple sclerosis revealed by optical coherence tomography and ophthalmoscopy
- Chapter 16 Optical coherence tomography and optic nerve magnetic resonance imaging in demyelinating diseases
- Chapter 17 Optical coherence tomography in neurologic clinical trials
- Chapter 18 Optical coherence tomography in a multi-center setting: quality control issues
- Chapter 19 Future technological advances in optical coherence tomography
- Index
- References
Summary
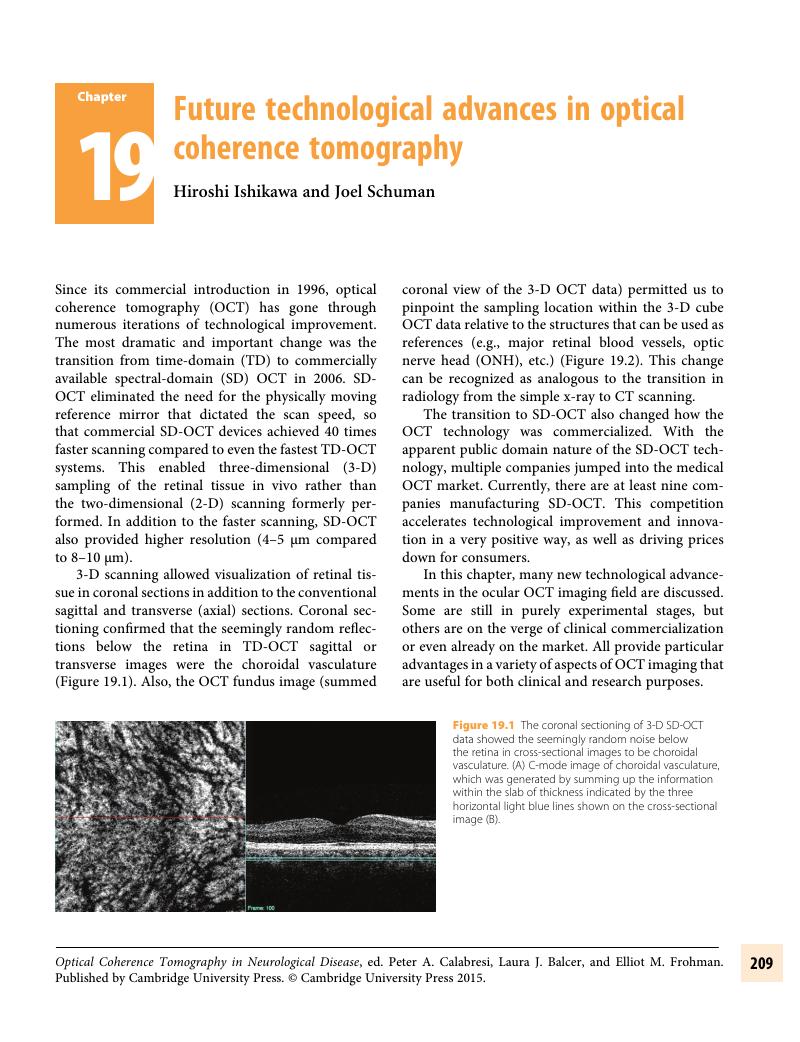
- Type
- Chapter
- Information
- Optical Coherence Tomography in Neurologic Diseases , pp. 209 - 217Publisher: Cambridge University PressPrint publication year: 2015