Pathologically exceeding mobilisation of non-esterified fatty acids (NEFA) from adipose tissue in the periparturient period is still of major concern regarding dairy health, welfare and productivity (McArt et al., Reference McArt, Nydam, Oetzel, Overton and Ospina2013). The necessity to mobilise metabolisable fuels out of body stores is caused by the disparity between dry matter intake (DMI) and energy expenditure via lactation referred to as negative energy balance (NEB). Besides NEB itself the individual cow's condition is an important factor influencing the extent of lipomobilisation (Vanholder et al., Reference Vanholder, Papen, Bemers, Vertenten and Berge2015). Body condition score (BCS) and DMI in turn are largely influenced by energetic feeding level and parity (Janovick and Drackley, Reference Janovick and Drackley2010; Janovick et al., Reference Janovick, Boisclair and Drackley2011). In rodents and humans there are remarkable disparities in functional properties of subcutaneous and visceral fat depots (Yang and Smith, Reference Yang and Smith2007) leading to dissimilarity in gain and loss and a varyingly strong impact on the development of metabolic disease (Bays, Reference Bays2014). In dairy cows, functional differences between adipose tissue depots (Locher et al., Reference Locher, Meyer, Weber, Rehage, Meyer, Dänicke and Huber2011; Saremi et al., Reference Saremi, Winand, Friedrichs, Kinoshita, Rehage, Dänicke, Haussler, Breves, Mielenz and Sauerwein2014) as well as asynchronicity of mobilisation and accumulation have been shown (von Soosten et al., Reference von Soosten, Meyer, Weber, Rehage, Flachowsky and Dänicke2011; Drackley et al., Reference Drackley, Wallace, Graugnard, Vasquez, Richards and Loor2014; Kabara et al., Reference Kabara, Sordillo, Holcombe and Contreras2014). Changes in BCS of the periparturient dairy cow and its possible impact on health and performance have been intensively studied in the last decades (Roche et al., Reference Roche, Friggens, Kay, Fisher, Stafford and Berry2009). However, comparatively little is known about the gain and loss of depot mass of different adipose tissues between late pregnancy and the first 100 days in milk (DIM).
Niacin is a potent antilipolytic agent applied in human and veterinary medicine. It is antagonistic to β-adrenergic activation of lipolysis and thereby reduces NEFA release from adipose tissue (Karpe and Frayn, Reference Karpe and Frayn2004). In periparturient dairy cows β-adrenergic activation is one important pathway triggering lipolysis (Jaster and Wegner, Reference Jaster and Wegner1981; Locher et al., Reference Locher, Meyer, Weber, Rehage, Meyer, Dänicke and Huber2011). Consequently, niacin has been successfully used in dairy cattle as an antilipolytic agent to lower plasma NEFA (Pires and Grummer, Reference Pires and Grummer2007). The present study aimed to estimate the mass of the respective adipose tissue depots at crucial time points in the period from drying off to 100 DIM and to describe possible impacts of parity, niacin supplementation and energy density in the ration. Further, it was intended to compare the gain and loss of depot mass in this period between the subcutaneous depot and the abdominal depot as a whole. By comparison of the abdominal subunits retroperitoneal, mesenteric and omental adipose tissue amongst each other, it should be clarified, whether these tissues act similarly or divergently concerning gain and loss of depot mass during this time span. In a last step, the individual mass gain of a certain depot during dry period should be linked to its individual loss until 100 DIM.
Materials and methods
The study comprised 47 healthy German Holsteins, between 1st and 4th lactation (heifers: n = 18, cows: n = 29), housed in a free stall housing system at the Institute of Animal Nutrition, Friedrich-Loeffler-Institut, Braunschweig, Germany. The trial started 42 d prior to the expected calving date (−42 DIM) and finished at 100 DIM. All procedures concerning treatment, handling and sampling were carried out in accordance with the German Animal Welfare Act (permit number: 33.9-42502-04-11/0444) pertaining to the protection of experimental animals and approved by the Lower Saxony State Office for Consumer Protection and Food Safety (LAVES). The feeding regime is described in detail by Tienken et al. (Reference Tienken, Kersten, Frahm, Meyer, Locher, Rehage, Huber, Kenez, Sauerwein, Mielenz and Dänicke2015). In brief it was intended to stimulate lipid mobilisation postpartum by varying the percentage of concentrate in the ration during dry period and during lactation. Further niacin was supplemented to study a possible antilipolytic effect of this substance. More details are given in the online Supplementary File (see S1.1). Ultrasonography of subcutaneous and retroperitoneal fat layers and body size measurements were performed at −42 DIM and 3, 21 and 100 DIM. For detailed description of the procedure see Raschka et al. (Reference Raschka, Ruda, Wenning, von Stemm, Pfarrer, Huber, Meyer, Dänicke and Rehage2016).
Data processing and statistical analysis
The estimated depot mass (eDM, kg) of subcutaneous adipose tissue (SCAT), abdominal adipose tissue as a whole (AAT) and its subunits retroperitoneal adipose tissue (RPAT), omental adipose tissue (OMAT) and mesenteric adipose tissue (MAT) was calculated using the equations described in Raschka et al. (Reference Raschka, Ruda, Wenning, von Stemm, Pfarrer, Huber, Meyer, Dänicke and Rehage2016). This method uses a multiple linear regression approach to estimate actual depot mass on the basis of ultrasonographic measurements of certain subcutaneous and retroperitoneal fat layers and selected body size measures. Root mean square error (RMSE) of the obtained equations is 3.4 kg for SCAT, 6.1 kg for AAT, 1.7 kg for RPAT, 3.2 kg for OMAT and 1.6 kg for MAT. The coefficients of determination (R 2) of correlations between predicted and actual weight are 0.85 for SCAT, 0.98 for AAT, 0.93 for RPAT, 0.84 for OMAT and 0.93 for MAT. Since the actual dry period differed between individuals, averages of daily gain (adG) and loss (adL) were calculated for dry period (−42 DIM to 3 DIM), fresh cow period (3 to 21 DIM) and early lactation period (21 and 100 DIM). Relative (rel) gain (%/day) and loss within dry period, fresh cow period or early lactation period respectively were calculated setting the eDM at the preceding time point as 100%. This was supposed to provide an indicator of the intrinsic activity of a certain depot during the respective time span. Thereby comparability between all depots measured should be facilitated. For more details of the calculations see section S2.1 in the Supplementary File.
Feeding regime neither by energy density nor by niacin supplementation exerted any effect on the variables of interest. Further, these variables were not influenced by parity. No interactions between these factors were found. For detailed description of the statistical model and results see S2.2. and tables S1 and S2a–c in the Supplementary File. Thus, the PROC MIXED of SAS 9.3 was used for the creation of a model with the factors time, parity and depot as fixed factors and cow as random factor. The model was initially run for SCAT and AAT and in a second step separately for the abdominal subunits RPAT, OMAT and MAT. To compare adG during dry period between depots, a paired t-test for SCAT and AAT and a one-way ANOVA with repeated measures for RPAT, OMAT and MAT were performed. The adL in fresh cow period and early lactation period were analysed by a two way ANOVA with repeated measures with the factors time and depot for SCAT and AAT and for RPAT, OMAT and MAT separately. Pearson's correlations of adG during dry period with adL in fresh cow period and adL in early lactation period as well as of adL in fresh cow period with adL in early lactation period were calculated for each depot separately. Results were considered significant with P < 0.05.
Results
The effect of time (P < 0.001) was reflected by an increase of adipose tissue mass during dry period and a decrease during fresh cow period and early lactation period in each depot. As a main effect of the factor depot, AAT could be shown to be bigger than SCAT (P < 0.001). Further there was an interaction (P < 0.001) between depot and time concerning SCAT and AAT (Table 1). The kind of interaction becomes obvious by the differences in adG and adL as depicted below (Figs. 1 and 2). The abdominal subunits did not differ between each other. However, the interaction between time and depot was also significant for RPAT, OMAT and MAT (Table 2). Parity again had no effect on the parameters of interest.
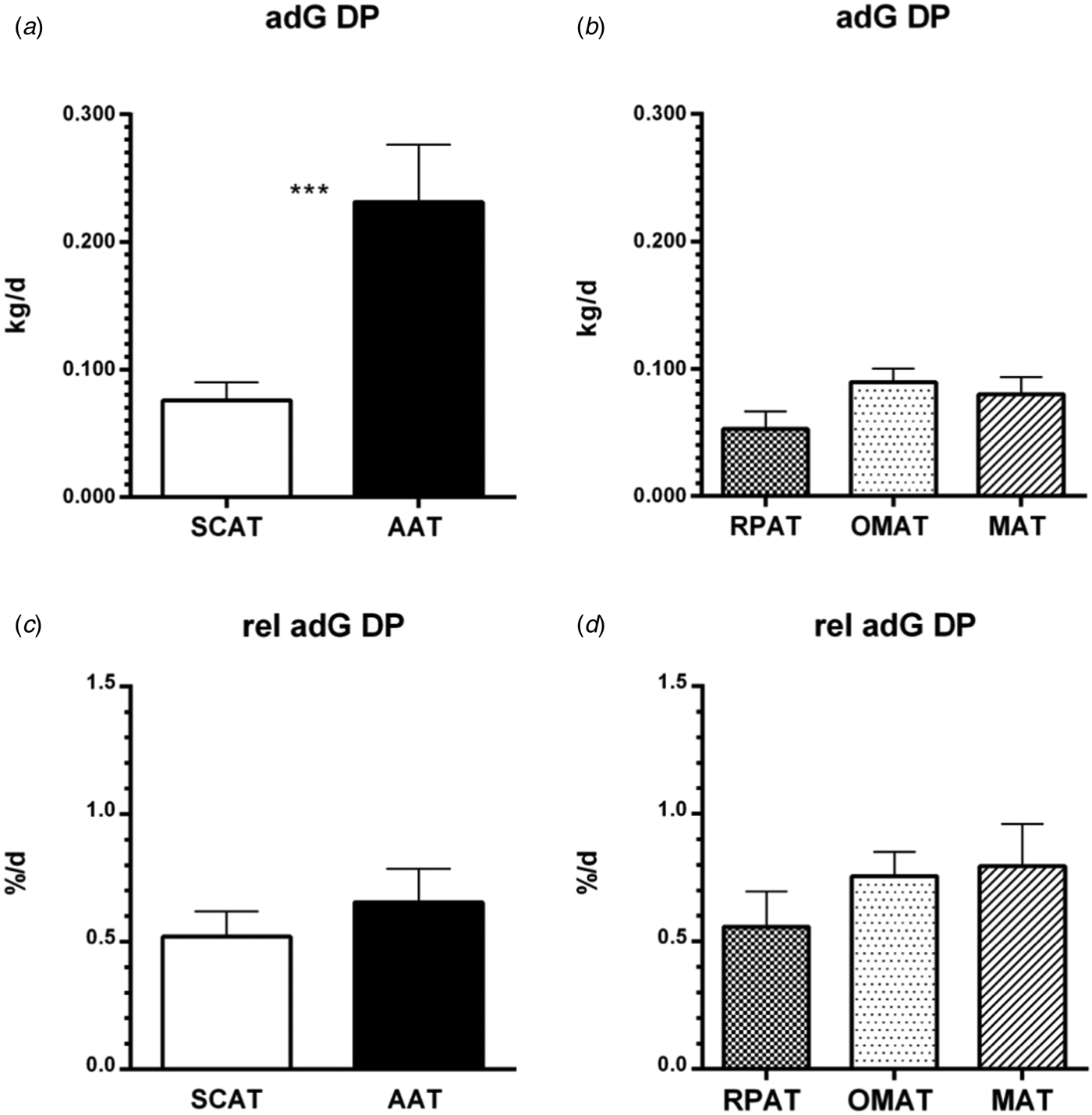
Fig. 1. Average and relative (rel) average daily gain (adG) of estimated depot mass (mean ± sem) in subcutaneous (SCAT), abdominal (AAT), retroperitoneal (RPAT), omental (OMAT) and mesenteric (MAT) adipose depot during dry cow period (DP; 42 d prior to expected calving to 3 DIM) in German Holstein heifers (n = 18) and cows (n = 29); *** significant difference between adjacent columns (P < 0.05)

Fig. 2. Average and relative (rel) average daily loss (adL) of estimated depot mass (mean ± sem) in subcutaneous (SCAT), abdominal (AAT), retroperitoneal (RPAT), omental (OMAT) and mesenteric (MAT) adipose depot during fresh cow period (FCP; 3 DIM to 21 DIM) and early lactation period (ELP; 22 DIM to 100 DIM) in German Holstein heifers (n = 18) and cows (n = 29); Asterisks indicate significant difference between columns. *P < 0.05; **P < 0.01; ***P < 0.001; ****P < 0.0001.
Table 1. Effects of depot (SCAT vs. AAT) and time (−42 to 100 DIM) on estimated adipose depot mass (kg) in German Holsteins (LSmeans ± se)

eDM, estimated depot mass; SCAT, subcutaneous adipose tissue; AAT, abdominal adipose tissue.
Table 2. Effects of depot (RPAT vs. OMAT vs. MAT) and time (−42 to 100 DIM) on estimated adipose depot mass (kg) in German Holsteins (LSmeans ± se)

eDM, estimated depot mass; RPAT, retroperitoneal adipose tissue; OMAT, omental adipose tissue; MAT, mesenteric adipose tissue.
In comparison to AAT, adG during dry period was significantly lower in SCAT (P = 0.001, Fig. 1a) whereas rel adG did not differ between these two depots (P = 0.1987, Fig. 1c). Concerning the abdominal subunits, adG and rel adG during dry period did not differ (Fig. 1b and d).
The adL (P < 0.0001) and rel adL (P = 0.0237) during fresh cow period were significantly lower in SCAT than in AAT but were not different in early lactation period (P > 0.05; Fig 2a and c). Intra-depot adL was higher in fresh cow period than in early lactation period (SCAT: P = 0.0001; AAT: P < 0.0001; Figure 2a). In OMAT adL in fresh cow period was lower than in MAT (P = 0.0045) whereas RPAT ranged in between and was not different from the other depots. This difference was levelled out in early lactation period (P > 0.05; Fig. 2b). Neither in fresh cow period nor in early lactation period did rel adL differ between abdominal subunits (Fig. 2d). Intra-depot adL in fresh cow period was higher than in early lactation period for RPAT (P < 0.0001), OMAT (P = 0.0011) and MAT (P < 0.0001; Fig. 2b).
Correlations between adG during dry period and adL in fresh cow period intra-depot were consistently significant (P < 0.001) and ranged from r = – 0.58 for SCAT to r = – 0.49 for AAT. The adG in dry cow period was significantly correlated with adL in early lactation period in OMAT (P < 0.05; r = – 0.34). The adLs in fresh cow period and early lactation period were not correlated in any of the depots. Details are given in Table S3 in the Supplementary File.
Discussion
All adipose depots showed the quantitative changes usually expected in periparturient dairy cows with an increase during dry period when energy balance is positive and a decrease in fresh cow period and early lactation period driven by NEB. Body composition measurements reported an average quantity of 42 to 54 kg of mobilised fat during NEB in dairy cows. Whereas Andrew et al. (Reference Andrew, Waldo and Erdman1994) reported 42.9 kg within the first 62.9 DIM, performing a direct chemical body composition analysis at slaughter, animals lost 54 kg between 14 d antepartum and 35 DIM in a study of Komaragiri and Erdman (Reference Komaragiri and Erdman1997) that was using the D2O dilution technique. Converted to a daily basis, animals in these studies lost about 0.6 and 1.1 kg of total body fat respectively. Animals in the present study lost about 1.1 kg per day in fresh cow period until 21 DIM (Fig. 2). Thus, assessment of body fat depots by ultrasonography was in similar range with direct chemical body composition measurements or D2O dilution technique.
As shown in the companion study by Tienken et al. (Reference Tienken, Kersten, Frahm, Meyer, Locher, Rehage, Huber, Kenez, Sauerwein, Mielenz and Dänicke2015) multiparous cows showed higher DMI and a more positive energy balance during the dry period than primiparous cows (P < 0.001). This effect was more pronounced in animals receiving a high percentage of concentrate during this time span (P < 0.005). In lactating animals only parity influenced DMI and energy balance; primiparous cows showed a lower DMI but a more positive energy balance than multiparous cows. Despite these differences no parity or concentrate effects on BCS and the estimated mass of adipose tissue stored in the different depots could be found in the present study. However, body weight was influenced by parity (Tienken et al., Reference Tienken, Kersten, Frahm, Meyer, Locher, Rehage, Huber, Kenez, Sauerwein, Mielenz and Dänicke2015). This indicates that in the setting of the present study the differences in body weight of about 12% that according to Tienken et al. (Reference Tienken, Kersten, Frahm, Meyer, Locher, Rehage, Huber, Kenez, Sauerwein, Mielenz and Dänicke2015) are driven by parity are mostly smaller than the individual variations in the amount of fat stored in a certain AT depot of a single animal. The reasons for the absence of an effect of niacin supplementation in the present study are also explicitly discussed by Tienken et al. (Reference Tienken, Kersten, Frahm, Meyer, Locher, Rehage, Huber, Kenez, Sauerwein, Mielenz and Dänicke2015). Even with comparatively high amounts of 24 g niacin per day, only nicotinamide but not niacin could be detected in the serum of the animals in the present study (data not shown). The antilipolytic effect of dietary niacin in bovine adipose tissue is exerted by niacin and not by nicotinamide (Kenéz et al., Reference Kenéz, Locher, Rehage, Dänicke and Huber2014). Thus the absence of niacin in the bloodstream of the experimental animals is considered the main reason for the lack of effects of a dietary niacin supplementation in the present study.
As shown in Table 1, the AAT depot as a whole was considerably bigger than the SCAT depot whereas the masses of the abdominal depots RPAT, OMAT and MAT did not differ amongst each other. Data of the present study are in accordance with previous studies of Raschka et al. (Reference Raschka, Ruda, Wenning, von Stemm, Pfarrer, Huber, Meyer, Dänicke and Rehage2016) and von Soosten et al. (Reference von Soosten, Meyer, Weber, Rehage, Flachowsky and Dänicke2011) that determined quantity of adipose tissue depots by carcass analysis in periparturient dairy cattle. However, in the study by von Soosten et al. (Reference von Soosten, Meyer, Weber, Rehage, Flachowsky and Dänicke2011) AAT is three to four times bigger than SCAT, whereas in the present study AAT was only two times bigger than SCAT. This difference might be due to the fact that von Soosten et al. (Reference von Soosten, Meyer, Weber, Rehage, Flachowsky and Dänicke2011) used primiparous cows whereas the present study comprised primi- and multiparous cows. Further von Soosten et al. (Reference von Soosten, Meyer, Weber, Rehage, Flachowsky and Dänicke2011) describe also RPAT, OMAT and MAT masses but don't give statistical analysis of differences between these depots. Nevertheless the mass of OMAT seemed to be numerically bigger than RPAT and MAT in that study. These differences might also be due to differences in the study protocols concerning the definition of the respective depots. In a study on nonpregnant and nonlactating cows, Drackley et al. (Reference Drackley, Wallace, Graugnard, Vasquez, Richards and Loor2014) found numerically similar amounts of mesenteric and omental adipose tissue but that study did not assess subcutaneous adipose tissue.
Higher adG during dry period in AAT compared with SCAT in combination with a similar rel adG during dry period becomes explicable by the higher amount of fat stored in AAT at −42 DIM (Table 1). If both depots grow by the same percentage per day, the absolute value of adG is necessarily bigger in AAT compared to SCAT. For the moment these data contradict a constitutionally higher adipogenic activity in AAT, at least during the dry period. Even though Weber et al. (Reference Weber, Locher, Huber, Kenéz, Rehage, Tienken, Meyer, Dänicke, Sauerwein and Mielenz2016a, Reference Weber, Locher, Huber, Rehage, Tienken, Meyer, Dänicke, Webb, Sauerwein and Mielenz2016b) in companion studies found higher mRNA expression of insulin dependent antilipolytic factors like hydroxycarboxylic receptor (HCAR) 1 and 2 in RPAT than in SCAT at −42 DIM, this did not lead to a relatively higher actual accretion of fat in AAT during dry period.
Several studies reveal discrepancies between changes in subcutaneous fat layers as represented by BCS, back fat thickness, fat depth or changes in body weight and biochemical markers of lipid mobilisation (NEFA and BHB). Therefore, it is often hypothesised that changes in internal or visceral adipose depots would be of greater importance in the context of lipomobilisation in dairy cows (Pires et al., Reference Pires, Delavaud, Faulconnier, Pomies and Chilliard2013) but unfortunately this is not reflected by common methods for the assessment of body condition. Not only the absolute weight of AAT depot (Table 1) but also adL in fresh cow period (Fig. 2a) was always bigger than in SCAT in our study. This supports the assumption that in times of NEB in fresh cow period quantitatively more fat is mobilised out of AAT than out of SCAT. Further, a higher intrinsic lipolytic activity is hypothesised for selected abdominal adipose tissues in the dairy cow (Locher et al., Reference Locher, Meyer, Weber, Rehage, Meyer, Dänicke and Huber2011; von Soosten et al., Reference von Soosten, Meyer, Weber, Rehage, Flachowsky and Dänicke2011; Kabara et al., Reference Kabara, Sordillo, Holcombe and Contreras2014). According to the present study this hypothesis could be confirmed for fresh cow period, because the relative adL in fresh cow period in AAT was also bigger than in SCAT (Fig. 2c). This higher intrinsic lipolytic activity seemed to be a property of AAT as a whole, for the abdominal subunits RPAT, OMAT and MAT despite higher adL during fresh cow period in MAT (Fig. 2b) did not differ in rel adL (Fig. 2d). Further, these differences between AAT and SCAT seemed to be diminished across lactation, for neither adL nor rel adL differed between depots during early lactation period (Fig. 2a,b). On the ultrastructural level this might become explicable by changes in morphology and functionality accompanying the depletion of fat cells during lipomobilisation. In dairy cattle, adipocyte size is considered to reflect, independently of its anatomic location, the actual ‘fill’ of a certain adipose depot (Locher et al., Reference Locher, Häussler, Laubenthal, Singh, Winkler, Kinoshita, Kenéz, Rehage, Huber, Sauerwein and Dänicke2015; De Koster et al., Reference De Koster, Van den Broeck, Hulpio, Claeys, Van Eetvelde, Hermans, Hostens, Fievez and Opsomer2016). In a companion study Kenéz et al. (Reference Kenéz, Kulcsar, Kluge, Benbelkacem, Hansen, Locher, Meyer, Rehage, Dänicke and Huber2015) found bigger fat cells in RPAT than in SCAT at 3 DIM and a more pronounced cell size decrease in RPAT postpartum. Recently it could be further shown in dairy cattle for SCAT and OMAT that bigger fat cells have a higher basal lipolytic activity and are more reactive to lipolytic stimuli (De Koster et al. Reference De Koster, Van den Broeck, Hulpio, Claeys, Van Eetvelde, Hermans, Hostens, Fievez and Opsomer2016). Assuming that OMAT and MAT underwent the same ultrastructural changes as RPAT, the higher adL and rel adL in AAT might be due to bigger fat cells with higher lipolytic activity in this depot. This was further supported by the fact that in the present study differences disappeared after 21 DIM when cell size is not different between depots anymore (Kenéz et al., Reference Kenéz, Kulcsar, Kluge, Benbelkacem, Hansen, Locher, Meyer, Rehage, Dänicke and Huber2015). Furthermore, the mentioned companion studies of Weber et al. (Reference Weber, Locher, Huber, Kenéz, Rehage, Tienken, Meyer, Dänicke, Sauerwein and Mielenz2016a, Reference Weber, Locher, Huber, Rehage, Tienken, Meyer, Dänicke, Webb, Sauerwein and Mielenz2016b) again confirm higher lipolytic potential in RPAT compared to SCAT due to differences in mRNA and protein expression of several effectors of lipolytic pathways. In the present study, MAT showed the biggest adL during fresh cow period. In studies on non-pregnant non-lactating cows, a comparatively low lipogenic potential for MAT is postulated (Ji et al., Reference Ji, Drackley, Khan and Loor2014). Another study found this thesis confirmed by smaller adipocytes in MAT compared to OMAT and RPAT at the end of dry period (Depreester et al., Reference Depreester, De Koster, Van Poucke, Hostens, Van den Broeck, Peelman, Contreras and Opsomer2018). Following these argumentations, higher adL during fresh cow period in MAT might point to a comparatively higher propensity of this depot to loose fat mass stored during dry period. In most of the studies on visceral adipose tissue in the bovine species, fat samples were harvested in a slaughter process, reflecting a single time point for each individual. Due to technical reasons, only for RPAT repeated biopsy sampling in vivo has been performed (Locher et al., Reference Locher, Meyer, Weber, Rehage, Meyer, Dänicke and Huber2011). A cross sectional study on different adipose tissues in dairy cattle by von Soosten et al. (Reference von Soosten, Meyer, Weber, Rehage, Flachowsky and Dänicke2011) compares actual mass of the respective depots in heifers slaughtered at 1, 42 and 105 DIM. In this study significant changes over time are found only for RPAT, but not for OMAT and MAT. These considerable differences in study design and protocol limit comparability of the mentioned studies with the present data.
Based on our results we can summarise, that higher rel adL of AAT during fresh cow period might be partly explicable by differences in cellularity, mRNA and protein expression in RPAT whereas the underlying processes in OMAT and MAT in the context of lipomobilisation in fresh cow period remain unclear so far. In humans and rodents, visceral adipose tissue depots are postulated as the instant resource for maintenance of energy homoeostasis in times of energy surplus or deprivation (Romao et al. Reference Romao, Jin, He, McAllister and Guan2013; Fried et al. Reference Fried, Lee and Karastergiou2015). Higher deposition during dry period combined with higher actual and relative loss during fresh cow period pointed to a similar function for AAT in dairy cattle, too. This is supported by the fact that dairy breeds accumulate relatively more abdominal fat than beef breeds (Wright and Russel, Reference Wright and Russel1984). Metabolites like NEFA and glycerol mobilised out of the abdominal adipose depots are directly drained to the liver (Montague and O'Rahilly, Reference Montague and O'Rahilly2000), thereby providing an immediate energy flux to meet the energy demands of this organ. The energy demands of the liver in the periparturient cow are particularly high in dairy breeds, not only because the liver synthesises large amounts of glucose that is needed for lactose synthesis but also to accomplish its tasks concerning periparturient protein turnover and acute phase reactions (Bertoni et al., Reference Bertoni, Trevisi, Han and Bionaz2008; Bertoni and Trevisi, Reference Bertoni and Trevisi2013).
Correlations between adG during dry period and average daily loss during fresh cow period were significant in all depots and ranged between r = −0.49 and r = −0.58. Therefore, 24–34% of the total variation in the amount of fat mobilised in the fresh cow period may be attributable to the amount of fat stored during the dry period. It would appear that the more an individual accumulates fat in the dry period, the more it mobilises in fresh cow period. Since the size of bovine adipocytes increases with the increase of adipose mass in a certain depot (at least in the short term), it can be assumed that individuals in the present study that gained more fat in dry period had bigger fat cells at the beginning of fresh cow period. Bigger fat cells have a higher basal lipolytic activity and are more reactive to lipolytic stimuli (De Koster et al., Reference De Koster, Van den Broeck, Hulpio, Claeys, Van Eetvelde, Hermans, Hostens, Fievez and Opsomer2016).
Bigger fat cells might be the reason for the bigger adL during fresh cow period in individuals with higher adG during dry period. With the start of lactation, this relationship was lost in the largest part of the depots and only AAT showed a relationship of adG during dry period and adL in early lactation period. In addition adL during fresh cow period and adL in early lactation period were not correlated in any depot. This possibly indicates that higher lipolytic activity in adipose tissue in fatter cows was a transient and reversible effect that disappeared, when adipose tissue amount and thereby fat cell size decreased.
When assessing a dairy cow's condition, it should be taken into account, that the largest part of adipose tissue is stored in AAT and that this depot besides its mere quantity further appears to have a higher intrinsic lipolytic activity. Thus it can be assumed that a remarkable part of adipose derived metabolites and metabolic effectors interacting in physiological and deregulated adaptation to NEB origins from AAT. To clarify the impact of the abdominal subunits RPAT, OMAT and MAT, longitudinal studies linking biopsy sampling with adipose tissue weight over time would be necessary. Strong correlations between gain during dry period and loss in fresh cow period point to the special importance of the individual's body condition on adipose tissue mobilisation.
In conclusion, we have shown that the largest amount of fat mobilised in the fresh cow period origins from abdominal stores. This might impact the pattern of adipose derived metabolites and metabolic effectors interacting in physiological and deregulated adaptation to negative energy balance.
Supplementary material
The supplementary material for this article can be found at https://doi.org/10.1017/S0022029919000542
Acknowledgements
The authors thank the German Research Foundation (DFG, Bonn, Germany) for financial support. Further the technical assistance of Lea Fieguth and Anja Schacht and the coworkers of the Experimental Station of the Institute of Animal Nutrition Friedrich-Loeffler-Institut Braunschweig, Germany is gratefully acknowledged.