Book contents
- Coarse Grained Simulation and Turbulent Mixing
- Coarse Grained Simulation and Turbulent Mixing
- Copyright page
- Dedication
- Contents
- Contributors
- Preface
- Prologue
- Part I Fundamentals
- Part II Challenges
- Part III Complex Mixing Consequences
- 8 Shock Driven Turbulence
- 9 Laser Driven Turbulence in High Energy Density Physics and Inertial Confinement Fusion Experiments
- 10 Drive Asymmetry, Convergence, and the Origin of Turbulence in Inertial Confinement Fusion Implosions
- 11 Rayleigh–Taylor Driven Turbulence
- 12 Spray Combustion in Swirling Flow
- 13 Combustion in Afterburning Behind Explosive Blasts
- Epilogue
- Index
- Plate section
- References
13 - Combustion in Afterburning Behind Explosive Blasts
from Part III - Complex Mixing Consequences
Published online by Cambridge University Press: 05 June 2016
- Coarse Grained Simulation and Turbulent Mixing
- Coarse Grained Simulation and Turbulent Mixing
- Copyright page
- Dedication
- Contents
- Contributors
- Preface
- Prologue
- Part I Fundamentals
- Part II Challenges
- Part III Complex Mixing Consequences
- 8 Shock Driven Turbulence
- 9 Laser Driven Turbulence in High Energy Density Physics and Inertial Confinement Fusion Experiments
- 10 Drive Asymmetry, Convergence, and the Origin of Turbulence in Inertial Confinement Fusion Implosions
- 11 Rayleigh–Taylor Driven Turbulence
- 12 Spray Combustion in Swirling Flow
- 13 Combustion in Afterburning Behind Explosive Blasts
- Epilogue
- Index
- Plate section
- References
Summary
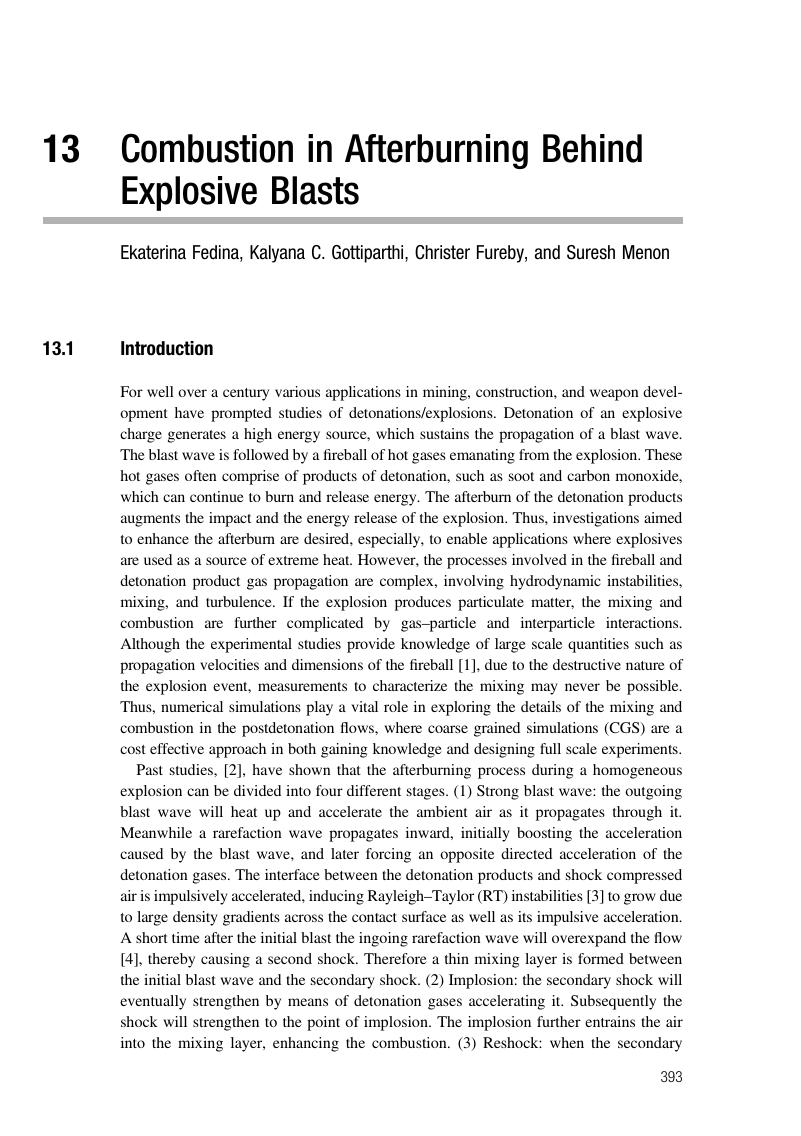
- Type
- Chapter
- Information
- Coarse Grained Simulation and Turbulent Mixing , pp. 393 - 431Publisher: Cambridge University PressPrint publication year: 2016
References
- 3
- Cited by