Book contents
- Radiogenic Isotope Geology
- Reviews
- Radiogenic Isotope Geology
- Copyright page
- Dedication
- Contents in Brief
- Contents
- Preface and Acknowledgements
- Chapter 1 Nucleosynthesis and Nuclear Decay
- Chapter 2 Mass Spectrometry
- Chapter 3 The Rb–Sr Method
- Chapter 4 The Sm–Nd Method
- Chapter 5 Lead Isotopes
- Chapter 6 Isotope Geochemistry of Oceanic Volcanics
- Chapter 7 Isotope Geochemistry of Continental Rocks
- Chapter 8 Osmium Isotopes
- Chapter 9 The Lu–Hf, Ba–La–Ce and K–Ca Systems
- Chapter 10 K–Ar, Ar–Ar and U–He Dating
- Chapter 11 Noble Gas Geochemistry
- Chapter 12 U-Series Dating
- Chapter 13 U-Series Geochemistry of Igneous Systems
- Chapter 14 Cosmogenic Nuclides
- Chapter 15 Extinct Radionuclides
- Chapter 16 Fission-Track Dating
- Book part
- Index
- References
Chapter 10 - K–Ar, Ar–Ar and U–He Dating
Published online by Cambridge University Press: 01 February 2018
- Radiogenic Isotope Geology
- Reviews
- Radiogenic Isotope Geology
- Copyright page
- Dedication
- Contents in Brief
- Contents
- Preface and Acknowledgements
- Chapter 1 Nucleosynthesis and Nuclear Decay
- Chapter 2 Mass Spectrometry
- Chapter 3 The Rb–Sr Method
- Chapter 4 The Sm–Nd Method
- Chapter 5 Lead Isotopes
- Chapter 6 Isotope Geochemistry of Oceanic Volcanics
- Chapter 7 Isotope Geochemistry of Continental Rocks
- Chapter 8 Osmium Isotopes
- Chapter 9 The Lu–Hf, Ba–La–Ce and K–Ca Systems
- Chapter 10 K–Ar, Ar–Ar and U–He Dating
- Chapter 11 Noble Gas Geochemistry
- Chapter 12 U-Series Dating
- Chapter 13 U-Series Geochemistry of Igneous Systems
- Chapter 14 Cosmogenic Nuclides
- Chapter 15 Extinct Radionuclides
- Chapter 16 Fission-Track Dating
- Book part
- Index
- References
Summary
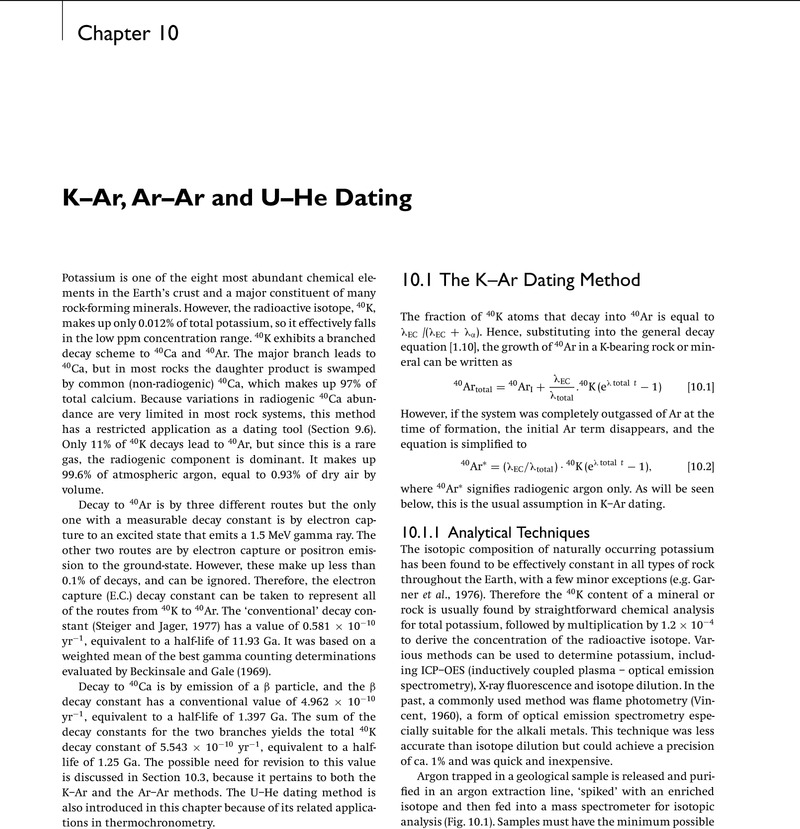
- Type
- Chapter
- Information
- Radiogenic Isotope Geology , pp. 240 - 273Publisher: Cambridge University PressPrint publication year: 2018