Book contents
- Ship Resistance and PropulsionSecond Edition
- Ship Resistance and Propulsion
- Copyright page
- Contents
- Preface to the Second Edition
- Preface to the First Edition
- Nomenclature
- Abbreviations
- Figure Acknowledgements
- 1 Introduction
- 2 Propulsive Power
- 3 Components of Hull Resistance
- 4 Model–Ship Extrapolation
- 5 Model–Ship Correlation
- 6 Restricted Water Depth and Breadth
- 7 Measurement of Resistance Components
- 8 Wake and Thrust Deduction
- 9 Numerical Estimation of Ship Resistance
- 10 Resistance Design Data
- 11 Propulsor Types
- 12 Propeller Characteristics
- 13 Powering Process
- 14 Hull Form Design
- 15 Numerical Methods for Propeller Analysis
- 16 Propulsor Design Data
- 17 Reductions in Propulsive Power and Emissions
- 18 Applications
- Book part
- Index
- References
15 - Numerical Methods for Propeller Analysis
Published online by Cambridge University Press: 25 August 2017
- Ship Resistance and PropulsionSecond Edition
- Ship Resistance and Propulsion
- Copyright page
- Contents
- Preface to the Second Edition
- Preface to the First Edition
- Nomenclature
- Abbreviations
- Figure Acknowledgements
- 1 Introduction
- 2 Propulsive Power
- 3 Components of Hull Resistance
- 4 Model–Ship Extrapolation
- 5 Model–Ship Correlation
- 6 Restricted Water Depth and Breadth
- 7 Measurement of Resistance Components
- 8 Wake and Thrust Deduction
- 9 Numerical Estimation of Ship Resistance
- 10 Resistance Design Data
- 11 Propulsor Types
- 12 Propeller Characteristics
- 13 Powering Process
- 14 Hull Form Design
- 15 Numerical Methods for Propeller Analysis
- 16 Propulsor Design Data
- 17 Reductions in Propulsive Power and Emissions
- 18 Applications
- Book part
- Index
- References
Summary
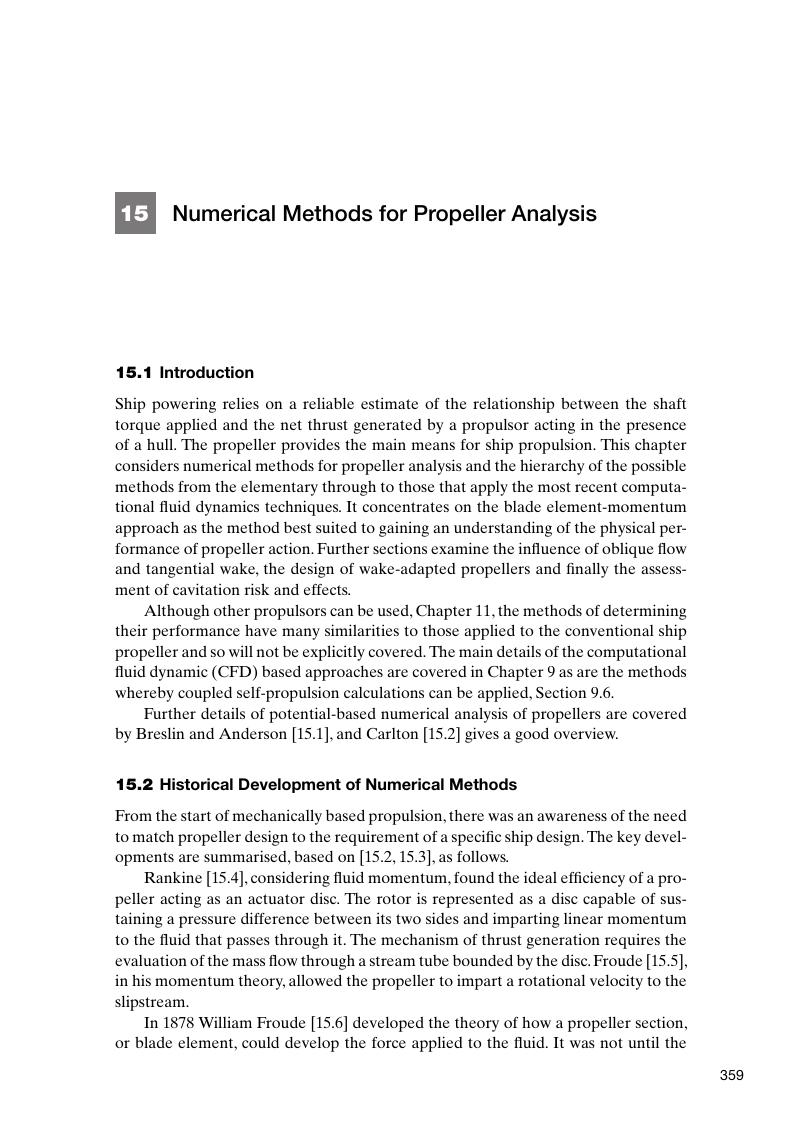
- Type
- Chapter
- Information
- Ship Resistance and PropulsionPractical Estimation of Ship Propulsive Power, pp. 359 - 394Publisher: Cambridge University PressPrint publication year: 2017