Book contents
- Frontmatter
- Contents
- Preface and Philosophy
- Abbreviations and acronyms
- Part I Planetary perspective
- Part II Earth: the dynamic planet
- Part III Radial and lateral structure
- Part IV Sampling the Earth
- Part V Mineral physics
- Part VI Origin and evolution of the layers and blobs
- Part VII Energetics
- References and notes
- Appendix
- Index
- References
References and notes
Published online by Cambridge University Press: 05 June 2012
- Frontmatter
- Contents
- Preface and Philosophy
- Abbreviations and acronyms
- Part I Planetary perspective
- Part II Earth: the dynamic planet
- Part III Radial and lateral structure
- Part IV Sampling the Earth
- Part V Mineral physics
- Part VI Origin and evolution of the layers and blobs
- Part VII Energetics
- References and notes
- Appendix
- Index
- References
Summary
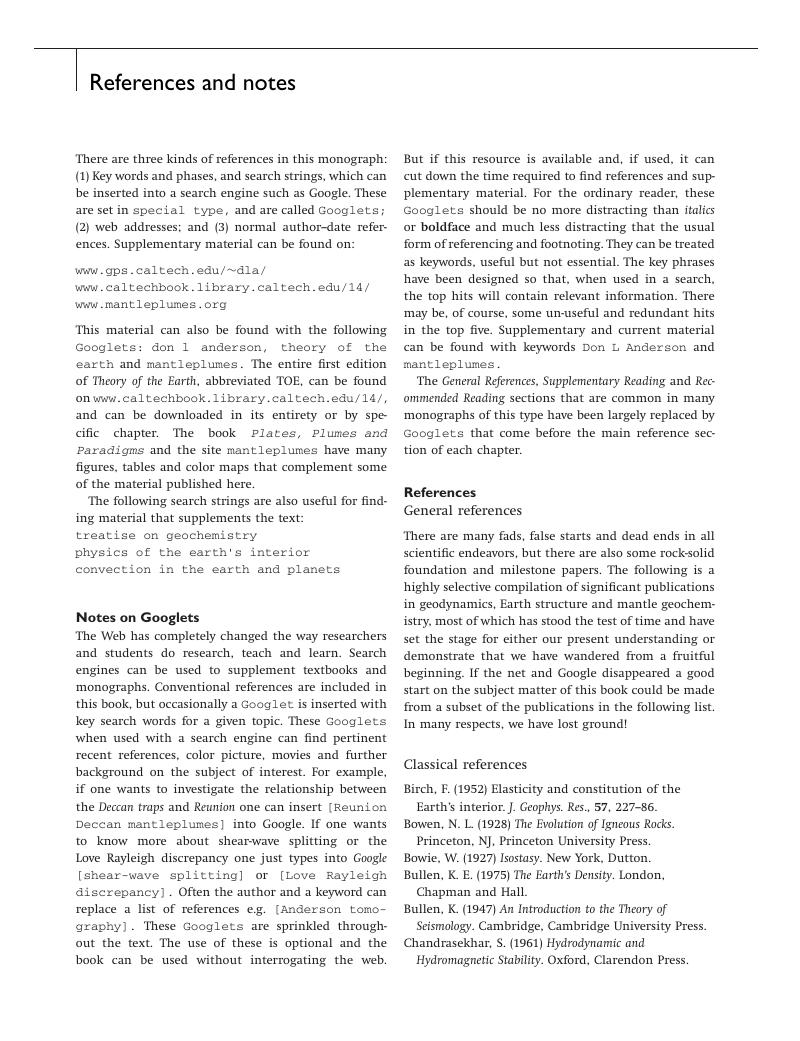
- Type
- Chapter
- Information
- New Theory of the Earth , pp. 356 - 374Publisher: Cambridge University PressPrint publication year: 2007
References
- 2
- Cited by