Book contents
- Frontmatter
- Contents
- List of contributors
- Preface
- Section 1 History and general issues
- Section 2 Cell biology and pathobiology
- 4 Immunophenotyping
- 5 Immunoglobulin and T-cell receptor gene rearrangements
- 6 Cytogenetics of acute leukemias
- 7 Molecular genetics of acute lymphoblastic leukemia
- 8 Molecular genetics of acute myeloid leukemia
- 9 Epigenetics of leukemia
- 10 Genetics and cellular drug resistance in acute leukemia
- 11 Heritable predisposition to childhood hematologic malignancies
- Section 3 Evaluation and treatment
- Section 4 Complications and supportive care
- Index
- Plate Section
- References
10 - Genetics and cellular drug resistance in acute leukemia
from Section 2 - Cell biology and pathobiology
Published online by Cambridge University Press: 05 April 2013
- Frontmatter
- Contents
- List of contributors
- Preface
- Section 1 History and general issues
- Section 2 Cell biology and pathobiology
- 4 Immunophenotyping
- 5 Immunoglobulin and T-cell receptor gene rearrangements
- 6 Cytogenetics of acute leukemias
- 7 Molecular genetics of acute lymphoblastic leukemia
- 8 Molecular genetics of acute myeloid leukemia
- 9 Epigenetics of leukemia
- 10 Genetics and cellular drug resistance in acute leukemia
- 11 Heritable predisposition to childhood hematologic malignancies
- Section 3 Evaluation and treatment
- Section 4 Complications and supportive care
- Index
- Plate Section
- References
Summary
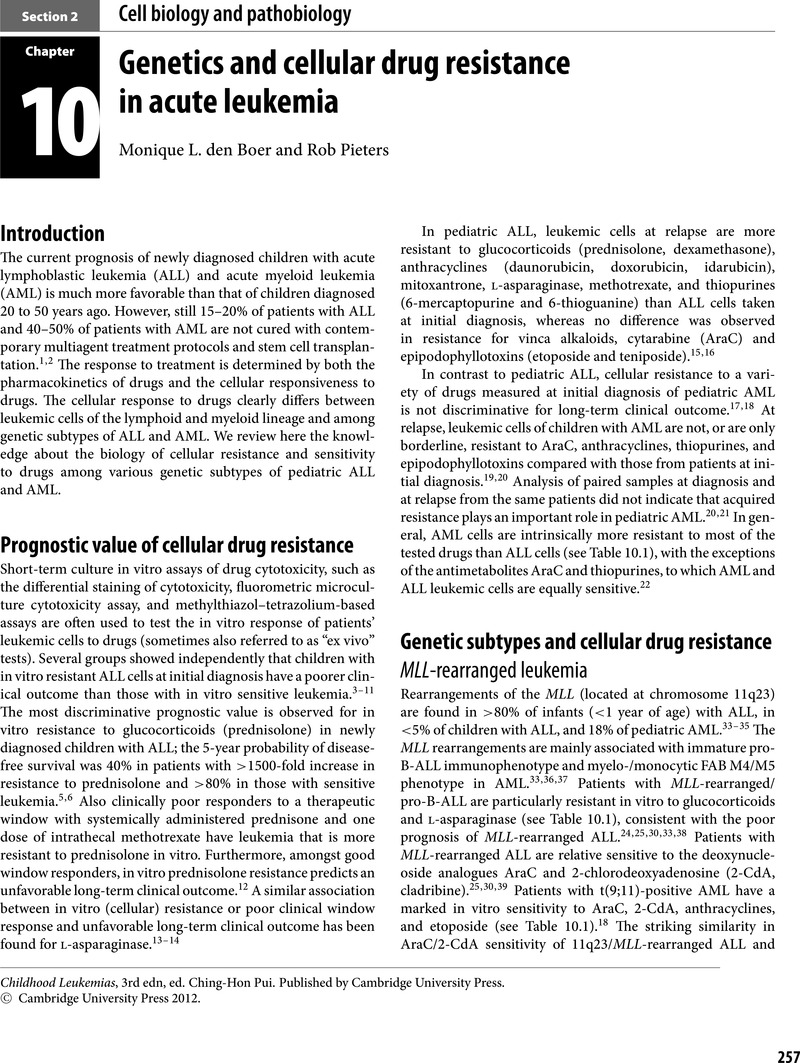
- Type
- Chapter
- Information
- Childhood Leukemias , pp. 257 - 275Publisher: Cambridge University PressPrint publication year: 2012
References
- 1
- Cited by