Book contents
- Fetal and Neonatal Brain Injury
- Fetal and Neonatal Brain Injury
- Copyright page
- Contents
- Contributors
- Preface
- Section 1 Epidemiology, Pathophysiology, and Pathogenesis of Fetal and Neonatal Brain Injury
- Section 2 Pregnancy, Labor, and Delivery Complications Causing Brain Injury
- Chapter 5 Prematurity and Complications of Labor and Delivery
- Chapter 6 Risks and Complications of Multiple Gestation
- Chapter 7 Intrauterine Growth Restriction
- Chapter 8 Maternal Diseases that Affect Fetal and Neonatal Neurodevelopment
- Chapter 9 Obstetric Conditions and Practices that Affect the Fetus and Newborn
- Chapter 10 Fetal and Neonatal Injury as a Consequence of Maternal Substance Abuse
- Chapter 11 Hypertensive Disorders of Pregnancy
- Chapter 12 Complications of Labor and Delivery
- Chapter 13 Fetal Responses to Asphyxia
- Chapter 14 Antepartum Evaluation of Fetal Well-Being
- Chapter 15 Intrapartum Evaluation of the Fetus
- Section 3 Diagnosis of the Infant with Brain Injury
- Section 4 Specific Conditions Associated with Fetal and Neonatal Brain Injury
- Index
- References
Chapter 13 - Fetal Responses to Asphyxia
from Section 2 - Pregnancy, Labor, and Delivery Complications Causing Brain Injury
Published online by Cambridge University Press: 13 December 2017
- Fetal and Neonatal Brain Injury
- Fetal and Neonatal Brain Injury
- Copyright page
- Contents
- Contributors
- Preface
- Section 1 Epidemiology, Pathophysiology, and Pathogenesis of Fetal and Neonatal Brain Injury
- Section 2 Pregnancy, Labor, and Delivery Complications Causing Brain Injury
- Chapter 5 Prematurity and Complications of Labor and Delivery
- Chapter 6 Risks and Complications of Multiple Gestation
- Chapter 7 Intrauterine Growth Restriction
- Chapter 8 Maternal Diseases that Affect Fetal and Neonatal Neurodevelopment
- Chapter 9 Obstetric Conditions and Practices that Affect the Fetus and Newborn
- Chapter 10 Fetal and Neonatal Injury as a Consequence of Maternal Substance Abuse
- Chapter 11 Hypertensive Disorders of Pregnancy
- Chapter 12 Complications of Labor and Delivery
- Chapter 13 Fetal Responses to Asphyxia
- Chapter 14 Antepartum Evaluation of Fetal Well-Being
- Chapter 15 Intrapartum Evaluation of the Fetus
- Section 3 Diagnosis of the Infant with Brain Injury
- Section 4 Specific Conditions Associated with Fetal and Neonatal Brain Injury
- Index
- References
Summary
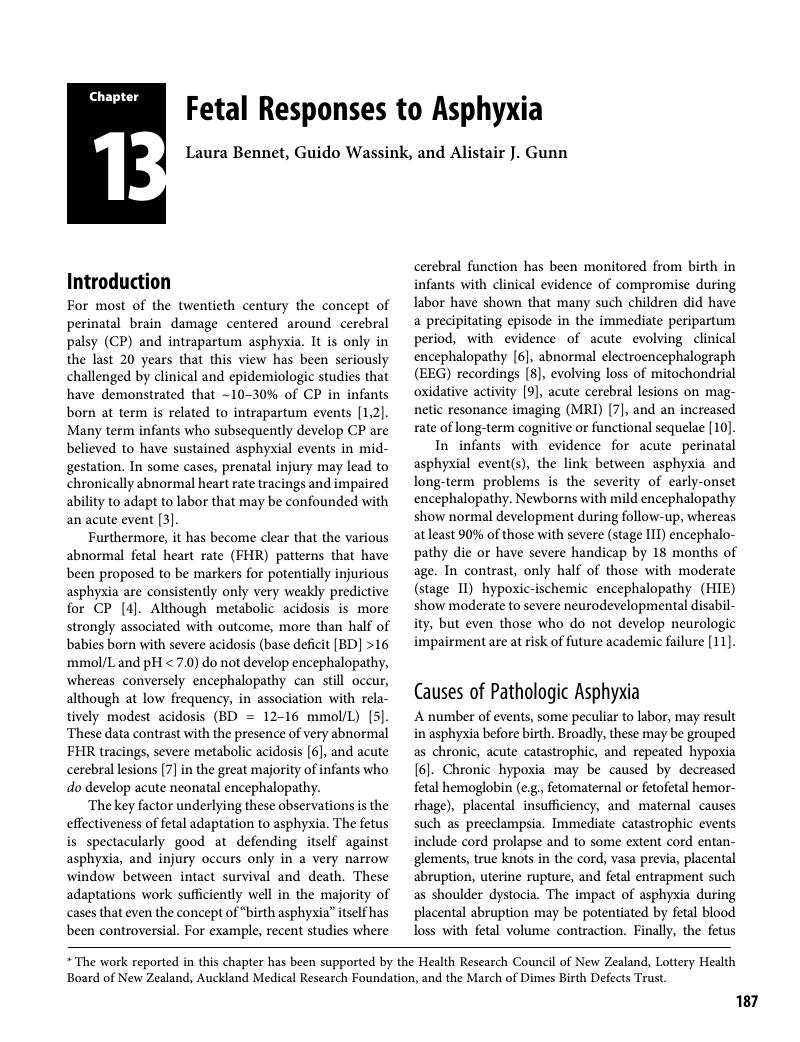
- Type
- Chapter
- Information
- Fetal and Neonatal Brain Injury , pp. 187 - 211Publisher: Cambridge University PressPrint publication year: 2017
References
- 1
- Cited by