Book contents
- The Behavioral Neurology of DementiaSecond Edition
- The Behavioral Neurology of Dementia
- Copyright page
- Contents
- Contributors
- Preface
- Chapter 1 Dementia at the bedside
- Chapter 2 Neuropsychiatry of dementia
- Chapter 3 Neuroimaging in dementia
- Chapter 4 Epidemiology and risk factors for dementia
- Chapter 5 Genetic approaches to neurodegenerative disease
- Chapter 6 Animal models of dementia
- Chapter 7 Neuropathology of dementia
- Chapter 8 Alzheimer’s disease
- Chapter 9 Frontotemporal dementia
- Chapter 10 Primary progressive aphasia
- Chapter 11 The non-fluent/agrammatic variant of primary progressive aphasia
- Chapter 12 Semantic dementia (semantic variant primary progressive aphasia)
- Chapter 13 Logopenic variant of primary progressive aphasia
- Chapter 14 The cognitive neurology of corticobasal degeneration and progressive supranuclear palsy
- Chapter 15 Amnestic mild cognitive impairment
- Chapter 16 Non-amnestic mild cognitive impairment
- Chapter 17 Early clinical features of the parkinsonian-related dementias
- Chapter 18 The Lewy body dementias
- Chapter 19 Cognitive and behavioral abnormalities of vascular cognitive impairment
- Chapter 20 CADASIL: a genetic model of arteriolar degeneration, white matter injury, and dementia in later life
- Chapter 21 Jakob–Creutzfeldt disease and other prion diseases
- Chapter 22 Autoimmune antibody-associated encephalopathy and dementia syndromes
- Chapter 23 Chronic traumatic encephalopathy
- Chapter 24 Treatment of Alzheimer’s disease
- Chapter 25 Cognitive disorders of the very old
- Chapter 26 Delirium masquerading as dementia
- Chapter 27 Sleep issues in dementia
- Index
- References
Chapter 5 - Genetic approaches to neurodegenerative disease
Published online by Cambridge University Press: 01 December 2016
- The Behavioral Neurology of DementiaSecond Edition
- The Behavioral Neurology of Dementia
- Copyright page
- Contents
- Contributors
- Preface
- Chapter 1 Dementia at the bedside
- Chapter 2 Neuropsychiatry of dementia
- Chapter 3 Neuroimaging in dementia
- Chapter 4 Epidemiology and risk factors for dementia
- Chapter 5 Genetic approaches to neurodegenerative disease
- Chapter 6 Animal models of dementia
- Chapter 7 Neuropathology of dementia
- Chapter 8 Alzheimer’s disease
- Chapter 9 Frontotemporal dementia
- Chapter 10 Primary progressive aphasia
- Chapter 11 The non-fluent/agrammatic variant of primary progressive aphasia
- Chapter 12 Semantic dementia (semantic variant primary progressive aphasia)
- Chapter 13 Logopenic variant of primary progressive aphasia
- Chapter 14 The cognitive neurology of corticobasal degeneration and progressive supranuclear palsy
- Chapter 15 Amnestic mild cognitive impairment
- Chapter 16 Non-amnestic mild cognitive impairment
- Chapter 17 Early clinical features of the parkinsonian-related dementias
- Chapter 18 The Lewy body dementias
- Chapter 19 Cognitive and behavioral abnormalities of vascular cognitive impairment
- Chapter 20 CADASIL: a genetic model of arteriolar degeneration, white matter injury, and dementia in later life
- Chapter 21 Jakob–Creutzfeldt disease and other prion diseases
- Chapter 22 Autoimmune antibody-associated encephalopathy and dementia syndromes
- Chapter 23 Chronic traumatic encephalopathy
- Chapter 24 Treatment of Alzheimer’s disease
- Chapter 25 Cognitive disorders of the very old
- Chapter 26 Delirium masquerading as dementia
- Chapter 27 Sleep issues in dementia
- Index
- References
Summary
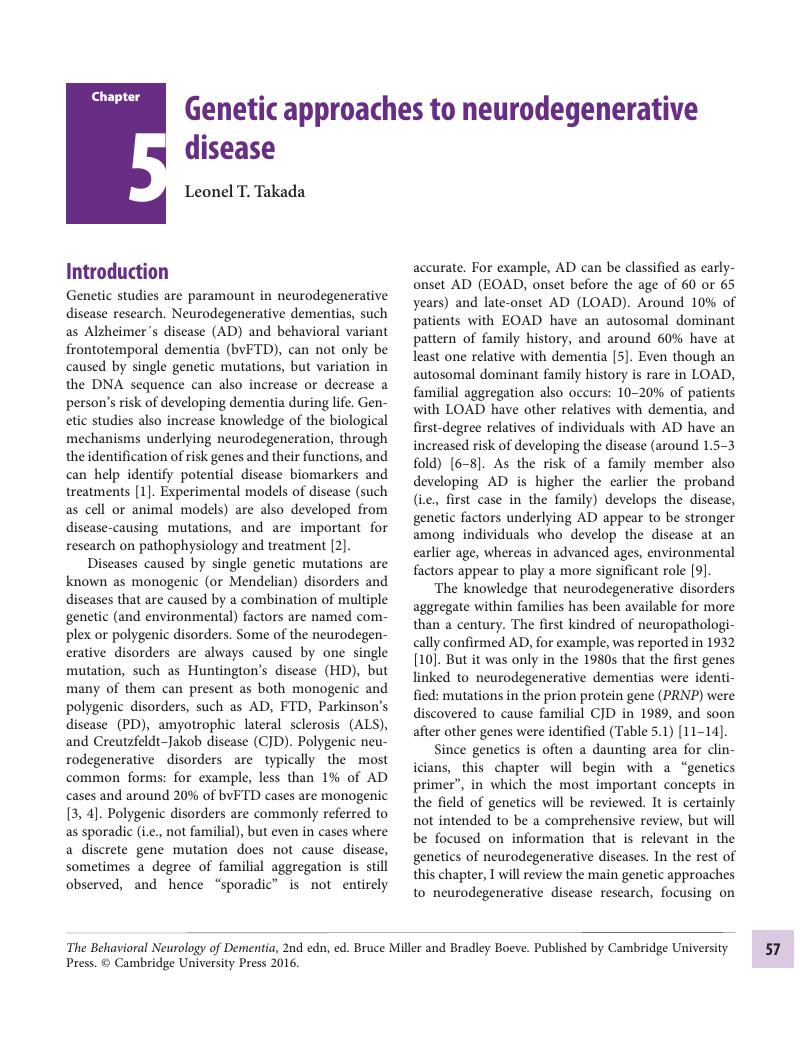
- Type
- Chapter
- Information
- The Behavioral Neurology of Dementia , pp. 57 - 76Publisher: Cambridge University PressPrint publication year: 2016