Book contents
- Frontmatter
- Contents
- Foreword by D. M. Engelman
- Foreword by Pierre Joliot
- Preface
- Introduction: Molecular biophysics at the beginning of the twenty-first century: from ensemble measurements to single-molecule detection
- Part A Biological macromolecules and physical tools
- Part B Mass spectrometry
- Part C Thermodynamics
- Part D Hydrodynamics
- Part E Optical spectroscopy
- Part F Optical microscopy
- Part G X-ray and neutron diffraction
- Part H Electron diffraction
- Part I Molecular dynamics
- Part J Nuclear magnetic resonance
- References
- Index of eminent scientists
- Subject Index
- References
References
Published online by Cambridge University Press: 05 November 2012
- Frontmatter
- Contents
- Foreword by D. M. Engelman
- Foreword by Pierre Joliot
- Preface
- Introduction: Molecular biophysics at the beginning of the twenty-first century: from ensemble measurements to single-molecule detection
- Part A Biological macromolecules and physical tools
- Part B Mass spectrometry
- Part C Thermodynamics
- Part D Hydrodynamics
- Part E Optical spectroscopy
- Part F Optical microscopy
- Part G X-ray and neutron diffraction
- Part H Electron diffraction
- Part I Molecular dynamics
- Part J Nuclear magnetic resonance
- References
- Index of eminent scientists
- Subject Index
- References
Summary
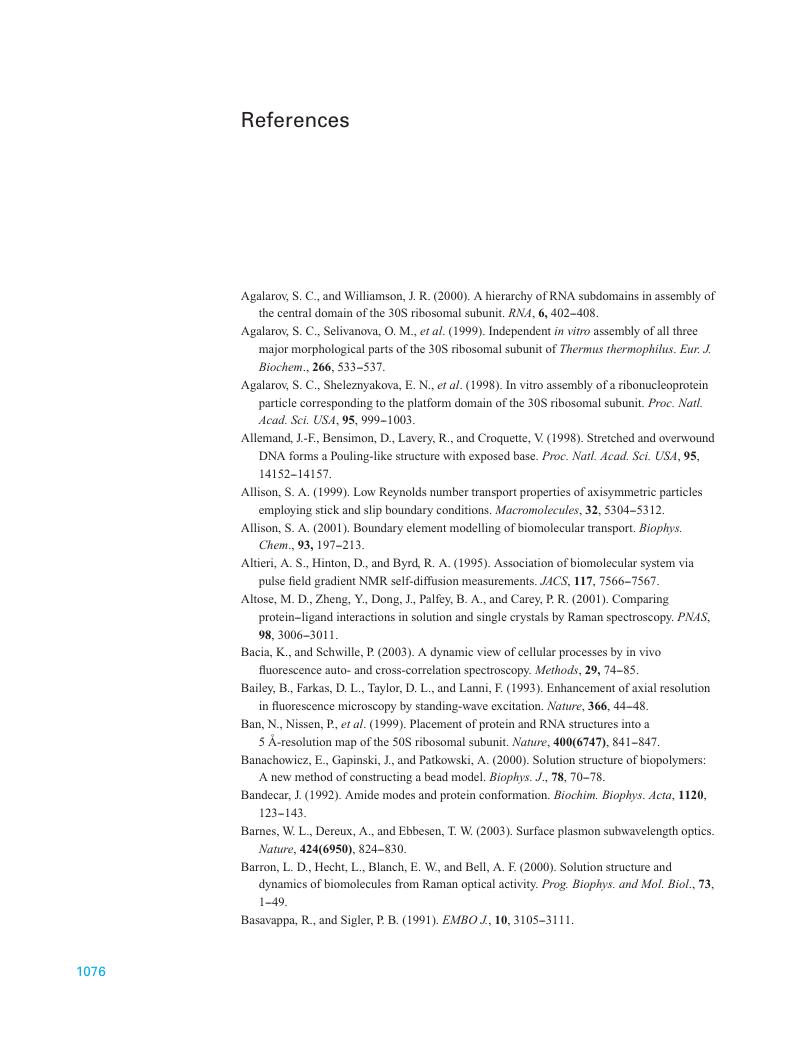
- Type
- Chapter
- Information
- Methods in Molecular BiophysicsStructure, Dynamics, Function, pp. 1076 - 1102Publisher: Cambridge University PressPrint publication year: 2007