Book contents
- Fetal and Neonatal Brain Injury
- Fetal and Neonatal Brain Injury
- Copyright page
- Contents
- Contributors
- Preface
- Section 1 Epidemiology, Pathophysiology, and Pathogenesis of Fetal and Neonatal Brain Injury
- Section 2 Pregnancy, Labor, and Delivery Complications Causing Brain Injury
- Section 3 Diagnosis of the Infant with Brain Injury
- Section 4 Specific Conditions Associated with Fetal and Neonatal Brain Injury
- Chapter 22 Congenital Malformations of the Brain
- Chapter 23 Neurogenetic Disorders of the Brain
- Chapter 24 Hemorrhagic Lesions of the Central Nervous System
- Chapter 25 Neonatal Stroke
- Chapter 26 Neurodevelopmental Consequences of Neonatal Hypoglycemia
- Chapter 27 Hyperbilirubinemia and Kernicterus
- Chapter 28 Polycythemia and Fetal-Maternal Bleeding
- Chapter 29 Hydrops Fetalis
- Chapter 30 Bacterial Sepsis in the Neonate
- Chapter 31 Neonatal Bacterial Meningitis
- Chapter 32 Neurologic Sequelae of Congenital and Perinatal Infections
- Chapter 33 Neurologic Complications of Perinatal Human Immunodeficiency Virus Infection
- Chapter 34 Inborn Errors of Metabolism and Single-Gene Disorders with Features of Neonatal Encephalopathy
- Chapter 35 Acidosis and Alkalosis
- Chapter 36 Persistent Pulmonary Hypertension of the Newborn
- Chapter 37 Pediatric Cardiac Surgery
- Chapter 38 Neonatal Resuscitation
- Chapter 39 Improving Performance, Reducing Error, and Minimizing Risk in the Delivery Room
- Chapter 40 Extended Management Following Resuscitation
- Chapter 41 Endogenous and Exogenous Neuroprotective Mechanisms after Hypoxic-Ischemic Injury
- Chapter 42 Neonatal Seizures
- Chapter 43 Nutritional Support of the Asphyxiated Infant
- Chapter 44 Early Outcomes after Extremely Preterm Birth
- Chapter 45 Cerebral Palsy
- Chapter 46 Neurodevelopmental and Neurobehavioral Outcomes of Prematurity
- Chapter 47 Neurocognitive Outcomes in Term Infants with Neonatal Encephalopathy
- Chapter 48 Neonatal Neuroethics
- Chapter 49 Medicolegal Issues in Perinatal Brain Injury
- Index
- References
Chapter 36 - Persistent Pulmonary Hypertension of the Newborn
from Section 4 - Specific Conditions Associated with Fetal and Neonatal Brain Injury
Published online by Cambridge University Press: 13 December 2017
- Fetal and Neonatal Brain Injury
- Fetal and Neonatal Brain Injury
- Copyright page
- Contents
- Contributors
- Preface
- Section 1 Epidemiology, Pathophysiology, and Pathogenesis of Fetal and Neonatal Brain Injury
- Section 2 Pregnancy, Labor, and Delivery Complications Causing Brain Injury
- Section 3 Diagnosis of the Infant with Brain Injury
- Section 4 Specific Conditions Associated with Fetal and Neonatal Brain Injury
- Chapter 22 Congenital Malformations of the Brain
- Chapter 23 Neurogenetic Disorders of the Brain
- Chapter 24 Hemorrhagic Lesions of the Central Nervous System
- Chapter 25 Neonatal Stroke
- Chapter 26 Neurodevelopmental Consequences of Neonatal Hypoglycemia
- Chapter 27 Hyperbilirubinemia and Kernicterus
- Chapter 28 Polycythemia and Fetal-Maternal Bleeding
- Chapter 29 Hydrops Fetalis
- Chapter 30 Bacterial Sepsis in the Neonate
- Chapter 31 Neonatal Bacterial Meningitis
- Chapter 32 Neurologic Sequelae of Congenital and Perinatal Infections
- Chapter 33 Neurologic Complications of Perinatal Human Immunodeficiency Virus Infection
- Chapter 34 Inborn Errors of Metabolism and Single-Gene Disorders with Features of Neonatal Encephalopathy
- Chapter 35 Acidosis and Alkalosis
- Chapter 36 Persistent Pulmonary Hypertension of the Newborn
- Chapter 37 Pediatric Cardiac Surgery
- Chapter 38 Neonatal Resuscitation
- Chapter 39 Improving Performance, Reducing Error, and Minimizing Risk in the Delivery Room
- Chapter 40 Extended Management Following Resuscitation
- Chapter 41 Endogenous and Exogenous Neuroprotective Mechanisms after Hypoxic-Ischemic Injury
- Chapter 42 Neonatal Seizures
- Chapter 43 Nutritional Support of the Asphyxiated Infant
- Chapter 44 Early Outcomes after Extremely Preterm Birth
- Chapter 45 Cerebral Palsy
- Chapter 46 Neurodevelopmental and Neurobehavioral Outcomes of Prematurity
- Chapter 47 Neurocognitive Outcomes in Term Infants with Neonatal Encephalopathy
- Chapter 48 Neonatal Neuroethics
- Chapter 49 Medicolegal Issues in Perinatal Brain Injury
- Index
- References
Summary
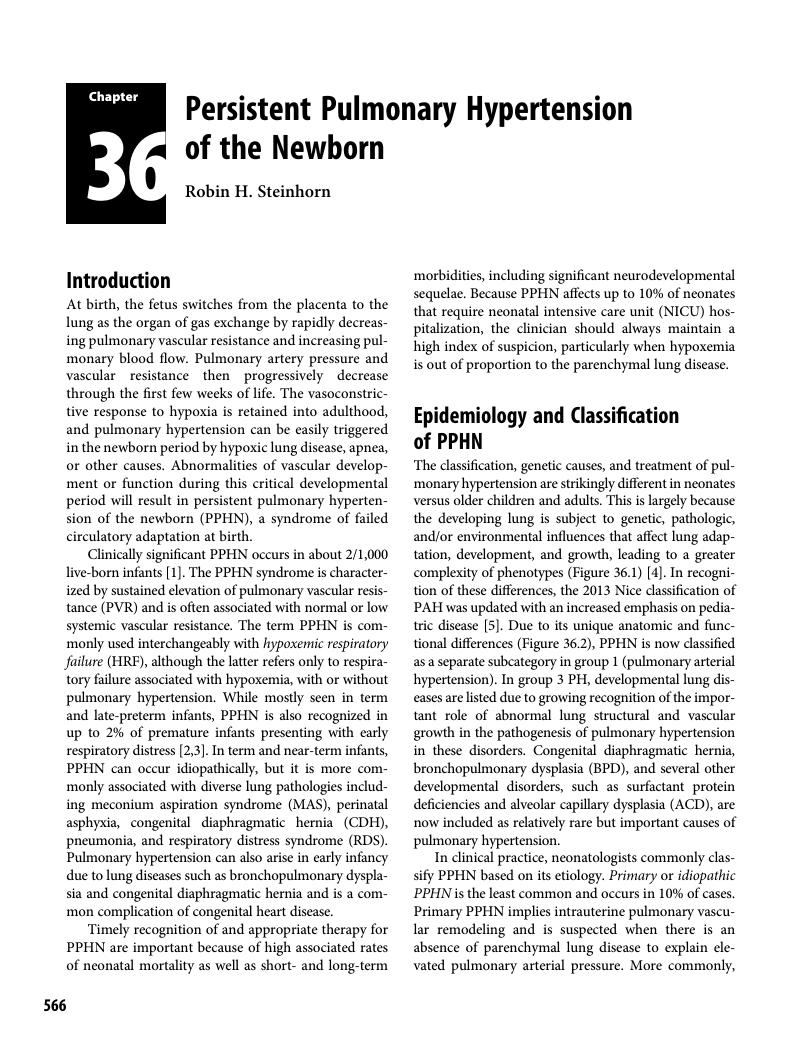
- Type
- Chapter
- Information
- Fetal and Neonatal Brain Injury , pp. 566 - 582Publisher: Cambridge University PressPrint publication year: 2017