Book contents
- The Atmosphere and Climate of Mars
- Cambridge Planetary Science
- The Atmosphere and Climate of Mars
- Copyright page
- Dedication
- Contents
- Contributors
- General Acknowledgments
- 1 Introduction
- 2 Understanding Mars and Its Atmosphere
- 3 History of Mars Atmosphere Observations
- 4 Thermal Structure and Composition
- 5 Mars Clouds
- 6 Radiative Process: Techniques and Applications
- 7 The Martian Planetary Boundary Layer
- 8 Mesoscale Meteorology
- 9 The Global Circulation
- 10 The Mars Dust Cycle
- 11 The Water Cycle
- 12 The CO2 Cycle
- 13 Atmospheric Photochemistry
- 14 Upper Neutral Atmosphere and Ionosphere
- 15 Solar Wind Interaction and Atmospheric Escape
- 16 Recent Climate Variations
- 17 The Early Mars Climate System
- 18 Future Prospects
- Index
- Plate section
- References
7 - The Martian Planetary Boundary Layer
Published online by Cambridge University Press: 05 July 2017
- The Atmosphere and Climate of Mars
- Cambridge Planetary Science
- The Atmosphere and Climate of Mars
- Copyright page
- Dedication
- Contents
- Contributors
- General Acknowledgments
- 1 Introduction
- 2 Understanding Mars and Its Atmosphere
- 3 History of Mars Atmosphere Observations
- 4 Thermal Structure and Composition
- 5 Mars Clouds
- 6 Radiative Process: Techniques and Applications
- 7 The Martian Planetary Boundary Layer
- 8 Mesoscale Meteorology
- 9 The Global Circulation
- 10 The Mars Dust Cycle
- 11 The Water Cycle
- 12 The CO2 Cycle
- 13 Atmospheric Photochemistry
- 14 Upper Neutral Atmosphere and Ionosphere
- 15 Solar Wind Interaction and Atmospheric Escape
- 16 Recent Climate Variations
- 17 The Early Mars Climate System
- 18 Future Prospects
- Index
- Plate section
- References
Summary
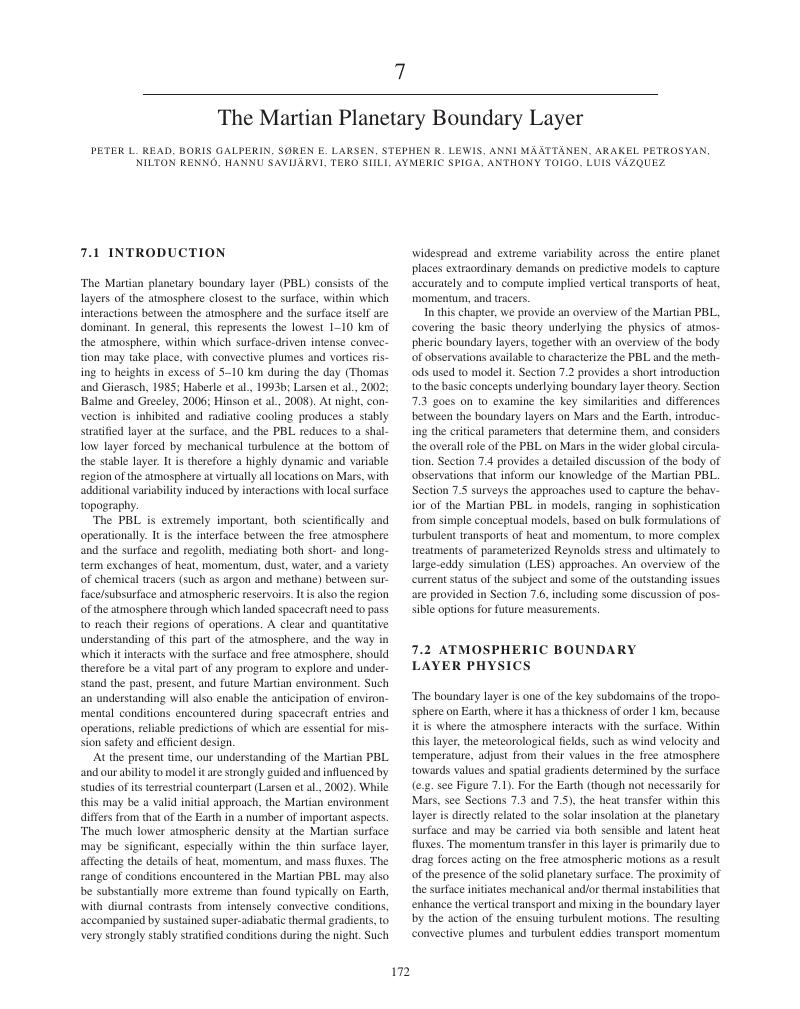
- Type
- Chapter
- Information
- The Atmosphere and Climate of Mars , pp. 172 - 202Publisher: Cambridge University PressPrint publication year: 2017
References
- 16
- Cited by