Section 2: - Fetal Disease: Pathogenesis and Treatment
Published online by Cambridge University Press: 21 October 2019
Summary
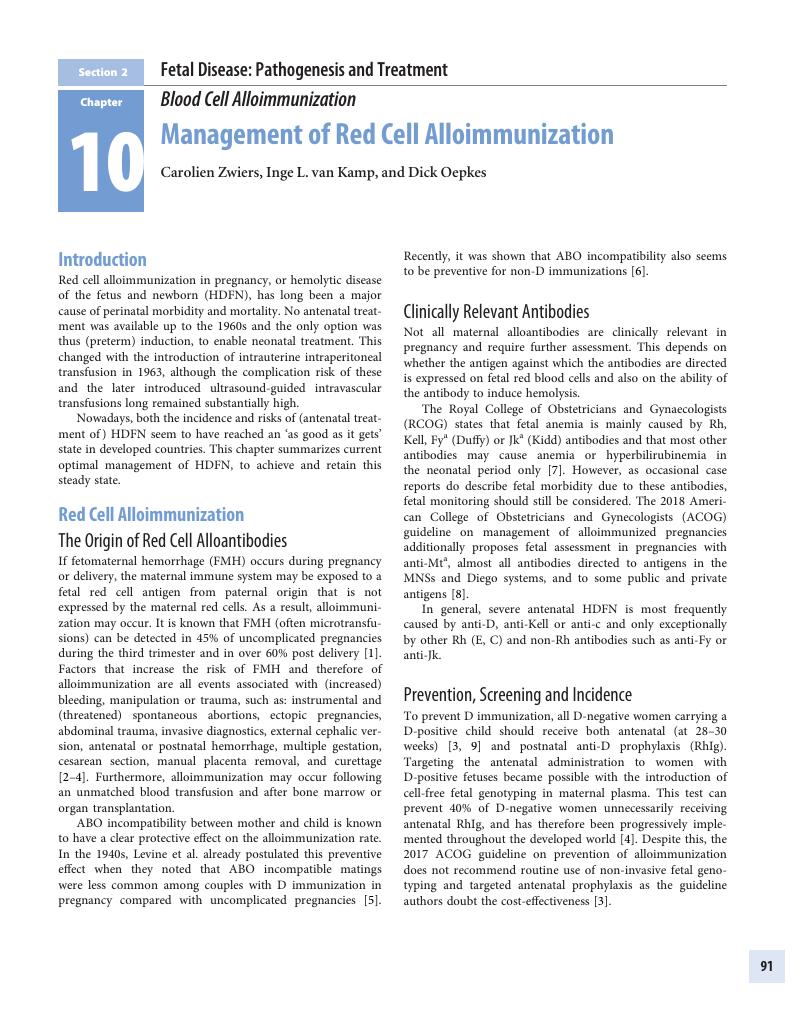
- Type
- Chapter
- Information
- Fetal TherapyScientific Basis and Critical Appraisal of Clinical Benefits, pp. 91 - 560Publisher: Cambridge University PressPrint publication year: 2020