Section 1: - General Principles
Published online by Cambridge University Press: 21 October 2019
Summary
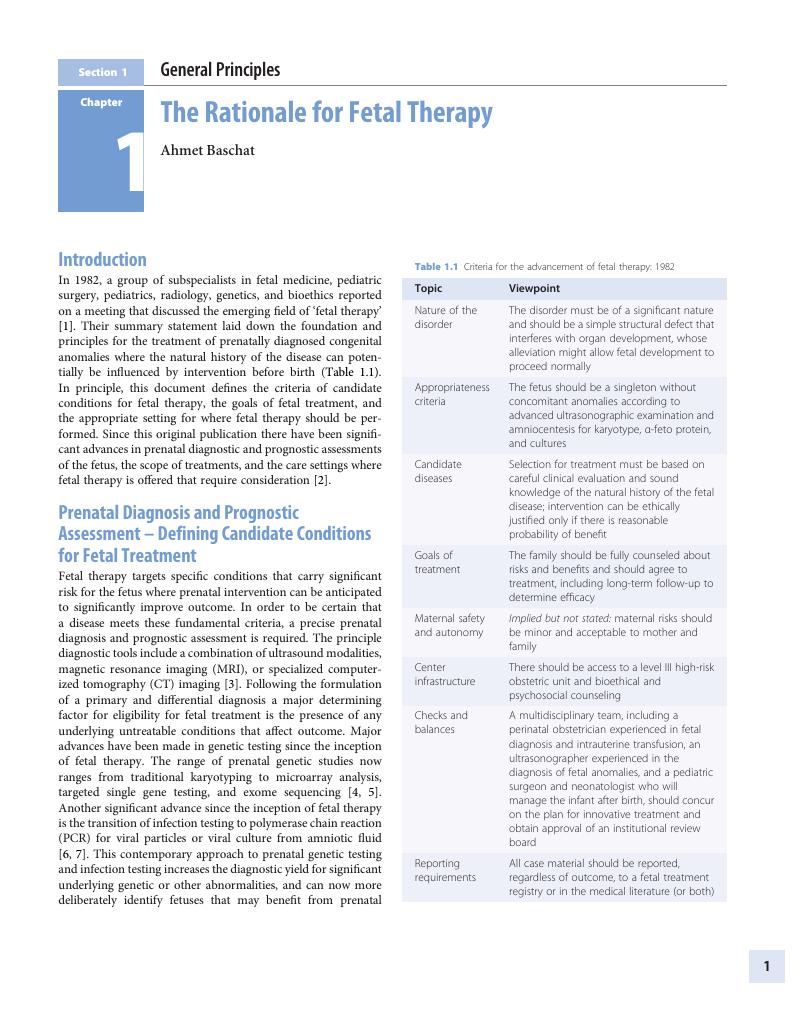
- Type
- Chapter
- Information
- Fetal TherapyScientific Basis and Critical Appraisal of Clinical Benefits, pp. 1 - 90Publisher: Cambridge University PressPrint publication year: 2020