Book contents
- Frontmatter
- Contents
- List of contributors
- Preface
- Part I Basic aspects of neurodegeneration
- 1 Endogenous free radicals and antioxidants in the brain
- 2 Biological oxidants and therapeutic antioxidants
- 3 Mitochondria, metabolic inhibitors and neurodegeneration
- 4 Excitoxicity and excitatory amino acid antagonists in chronic neurodegenerative diseases
- 5 Glutamate transporters
- 6 Calcium binding proteins in selective vulnerability of motor neurons
- 7 Apoptosis in neurodegenerative diseases
- 8 Neurotrophic factors
- 9 Protein misfolding and cellular defense mechanisms in neurodegenerative diseases
- 10 Neurodegenerative disease and the repair of oxidatively damaged DNA
- 11 Compounds acting on ion channels
- 12 The role of nitric oxide and PARP in neuronal cell death
- 13 Copper and zinc in Alzheimer's disease and amyotrophic lateral sclerosis
- 14 The role of inflammation in Alzheimer's disease neuropathology and clinical dementia. From epidemiology to treatment
- 15 Selected genetically engineered models relevant to human neurodegenerative disease
- 16 Toxic animal models
- 17 A genetic outline of the pathways to cell death in Alzheimer's disease, Parkinson's disease, frontal dementias and related disorders
- 18 Neurophysiology of Parkinson's disease, levodopa-induced dyskinesias, dystonia, Huntington's disease and myoclonus
- Part II Neuroimaging in neurodegeneration
- Part III Therapeutic approaches in neurodegeneration
- Normal aging
- Part IV Alzheimer's disease
- Part VI Other Dementias
- Part VII Parkinson's and related movement disorders
- Part VIII Cerebellar degenerations
- Part IX Motor neuron diseases
- Part X Other neurodegenerative diseases
- Index
- References
1 - Endogenous free radicals and antioxidants in the brain
from Part I - Basic aspects of neurodegeneration
Published online by Cambridge University Press: 04 August 2010
- Frontmatter
- Contents
- List of contributors
- Preface
- Part I Basic aspects of neurodegeneration
- 1 Endogenous free radicals and antioxidants in the brain
- 2 Biological oxidants and therapeutic antioxidants
- 3 Mitochondria, metabolic inhibitors and neurodegeneration
- 4 Excitoxicity and excitatory amino acid antagonists in chronic neurodegenerative diseases
- 5 Glutamate transporters
- 6 Calcium binding proteins in selective vulnerability of motor neurons
- 7 Apoptosis in neurodegenerative diseases
- 8 Neurotrophic factors
- 9 Protein misfolding and cellular defense mechanisms in neurodegenerative diseases
- 10 Neurodegenerative disease and the repair of oxidatively damaged DNA
- 11 Compounds acting on ion channels
- 12 The role of nitric oxide and PARP in neuronal cell death
- 13 Copper and zinc in Alzheimer's disease and amyotrophic lateral sclerosis
- 14 The role of inflammation in Alzheimer's disease neuropathology and clinical dementia. From epidemiology to treatment
- 15 Selected genetically engineered models relevant to human neurodegenerative disease
- 16 Toxic animal models
- 17 A genetic outline of the pathways to cell death in Alzheimer's disease, Parkinson's disease, frontal dementias and related disorders
- 18 Neurophysiology of Parkinson's disease, levodopa-induced dyskinesias, dystonia, Huntington's disease and myoclonus
- Part II Neuroimaging in neurodegeneration
- Part III Therapeutic approaches in neurodegeneration
- Normal aging
- Part IV Alzheimer's disease
- Part VI Other Dementias
- Part VII Parkinson's and related movement disorders
- Part VIII Cerebellar degenerations
- Part IX Motor neuron diseases
- Part X Other neurodegenerative diseases
- Index
- References
Summary
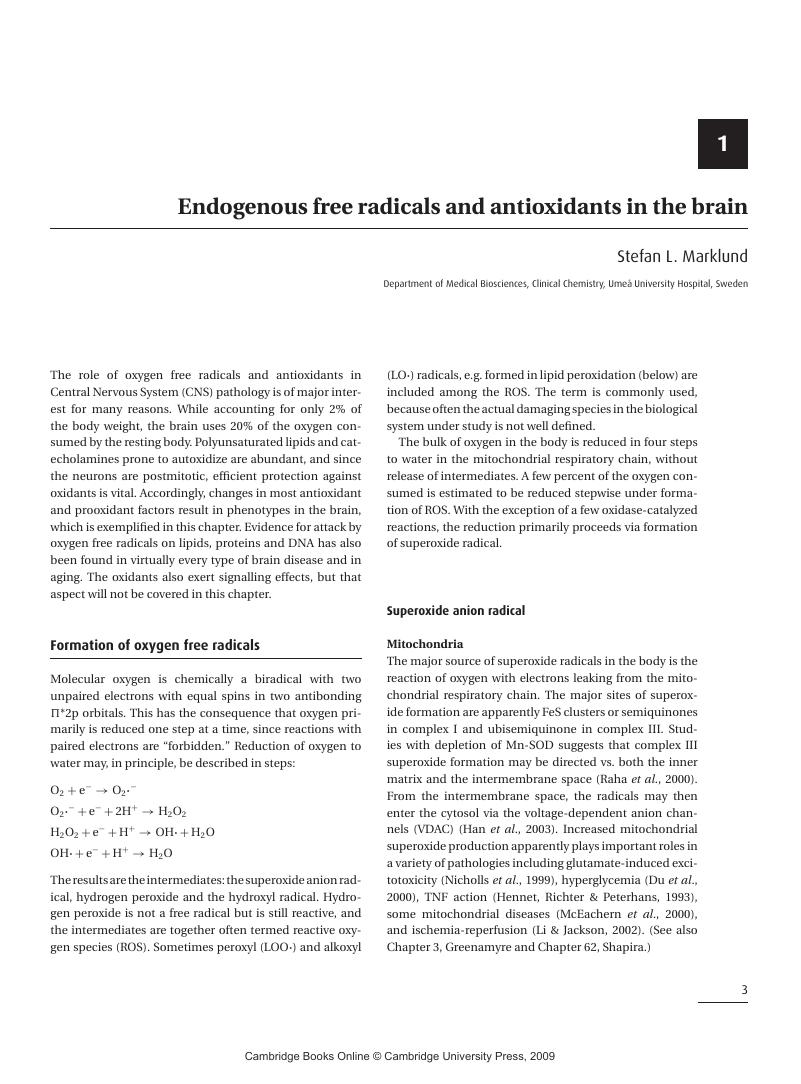
- Type
- Chapter
- Information
- Neurodegenerative DiseasesNeurobiology, Pathogenesis and Therapeutics, pp. 3 - 17Publisher: Cambridge University PressPrint publication year: 2005