References
Published online by Cambridge University Press: 05 June 2012
Summary
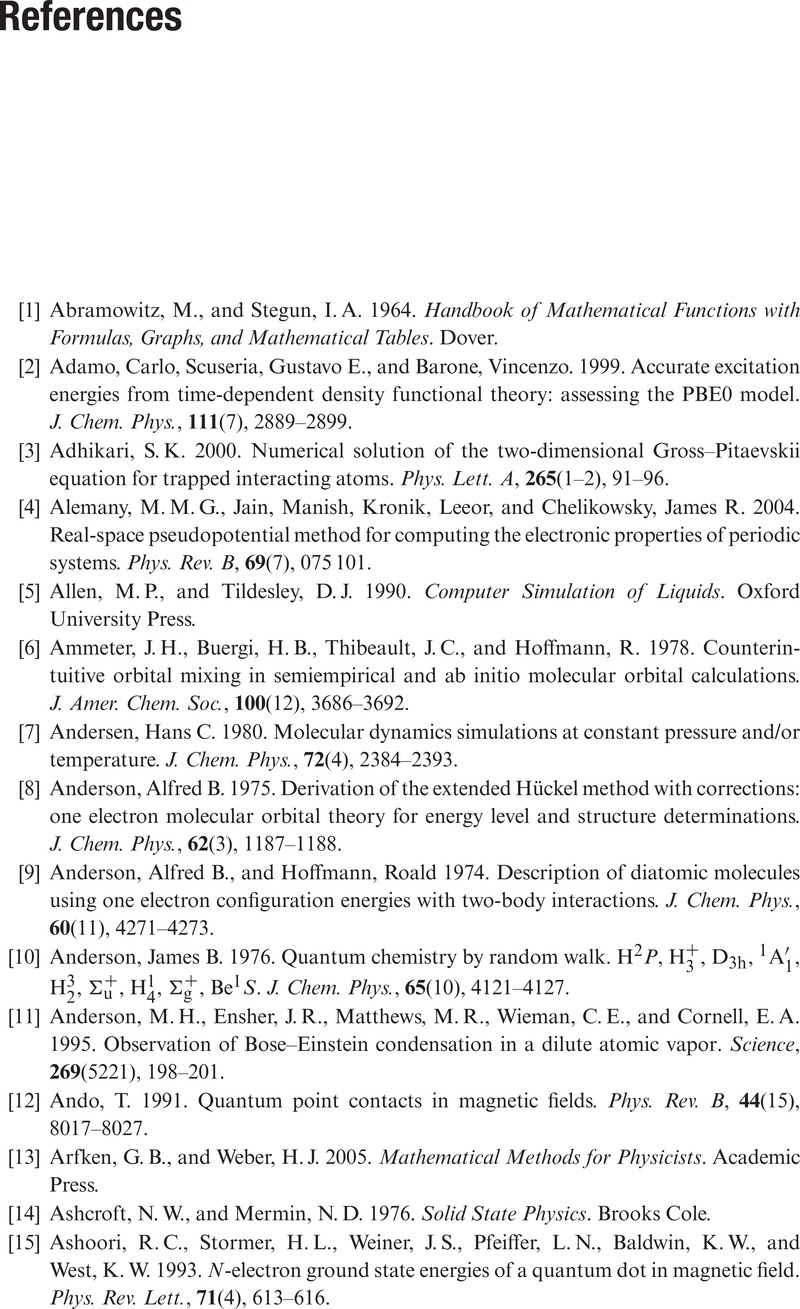
- Type
- Chapter
- Information
- Computational NanoscienceApplications for Molecules, Clusters, and Solids, pp. 409 - 427Publisher: Cambridge University PressPrint publication year: 2011