Book contents
- Frontmatter
- Contents
- List of contributors
- Preface
- Section 1 History and general issues
- Section 2 Cell biology and pathobiology
- Section 3 Evaluation and treatment
- Section 4 Complications and supportive care
- 29 Acute complications
- 30 Late complications after leukemia therapy
- 31 Therapy-related leukemias
- 32 Infectious complications in leukemia
- 33 Hematologic supportive care
- 34 Pain management
- 35 Psychosocial issues
- 36 Nursing care
- Index
- Plate Section
- References
31 - Therapy-related leukemias
from Section 4 - Complications and supportive care
Published online by Cambridge University Press: 05 April 2013
- Frontmatter
- Contents
- List of contributors
- Preface
- Section 1 History and general issues
- Section 2 Cell biology and pathobiology
- Section 3 Evaluation and treatment
- Section 4 Complications and supportive care
- 29 Acute complications
- 30 Late complications after leukemia therapy
- 31 Therapy-related leukemias
- 32 Infectious complications in leukemia
- 33 Hematologic supportive care
- 34 Pain management
- 35 Psychosocial issues
- 36 Nursing care
- Index
- Plate Section
- References
Summary
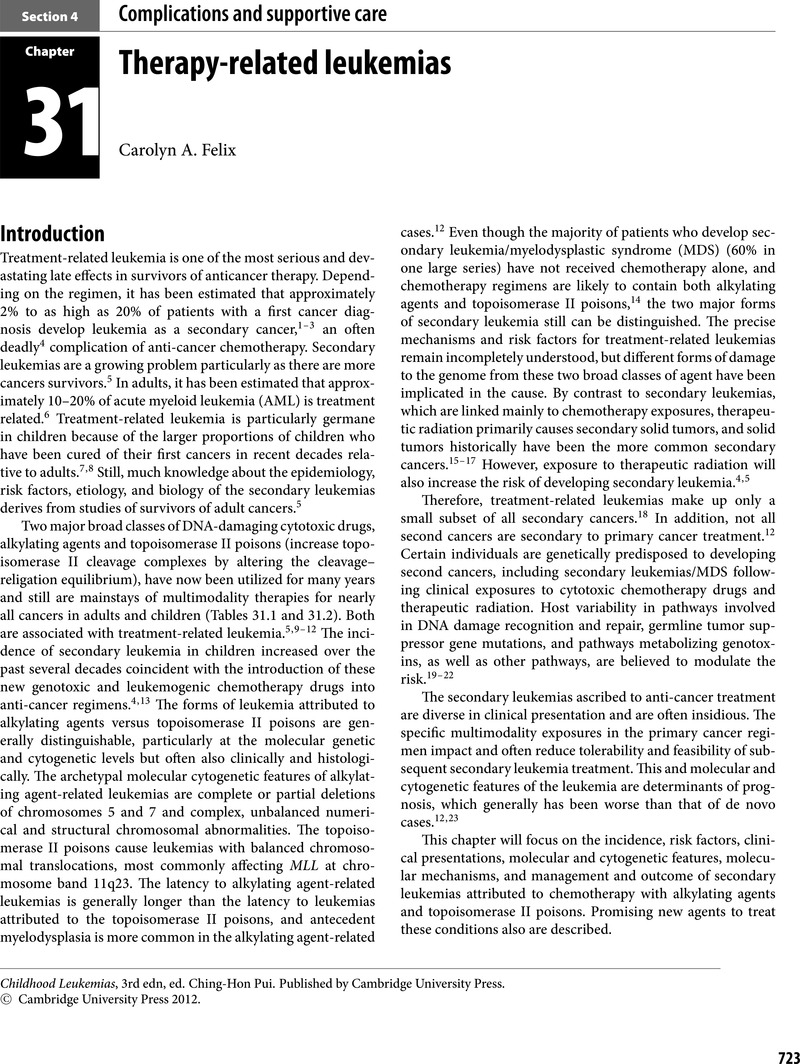
- Type
- Chapter
- Information
- Childhood Leukemias , pp. 723 - 771Publisher: Cambridge University PressPrint publication year: 2012
References
- 1
- Cited by