Part I - The science of icebergs
Published online by Cambridge University Press: 05 December 2015
Summary
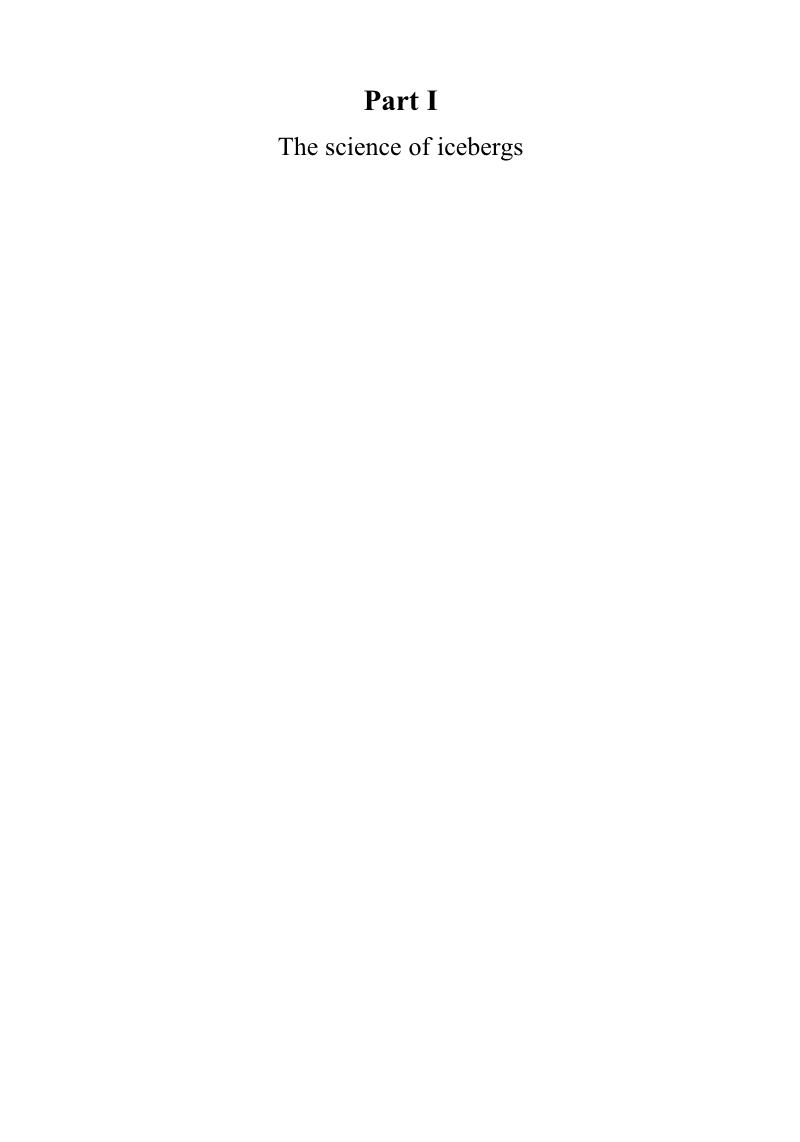
- Type
- Chapter
- Information
- IcebergsTheir Science and Links to Global Change, pp. 21 - 124Publisher: Cambridge University PressPrint publication year: 2015