Book contents
- Frontmatter
- Contents
- List of contributors
- Foreword
- Preface
- Section I Introduction
- Section II Data preparation
- Section III Phylogenetic inference
- Section IV Testing models and trees
- Section V Molecular adaptation
- Section VI Recombination
- Section VII Population genetics
- Section VIII Additional topics
- Glossary
- References
- Index
- References
References
Published online by Cambridge University Press: 05 June 2012
- Frontmatter
- Contents
- List of contributors
- Foreword
- Preface
- Section I Introduction
- Section II Data preparation
- Section III Phylogenetic inference
- Section IV Testing models and trees
- Section V Molecular adaptation
- Section VI Recombination
- Section VII Population genetics
- Section VIII Additional topics
- Glossary
- References
- Index
- References
Summary
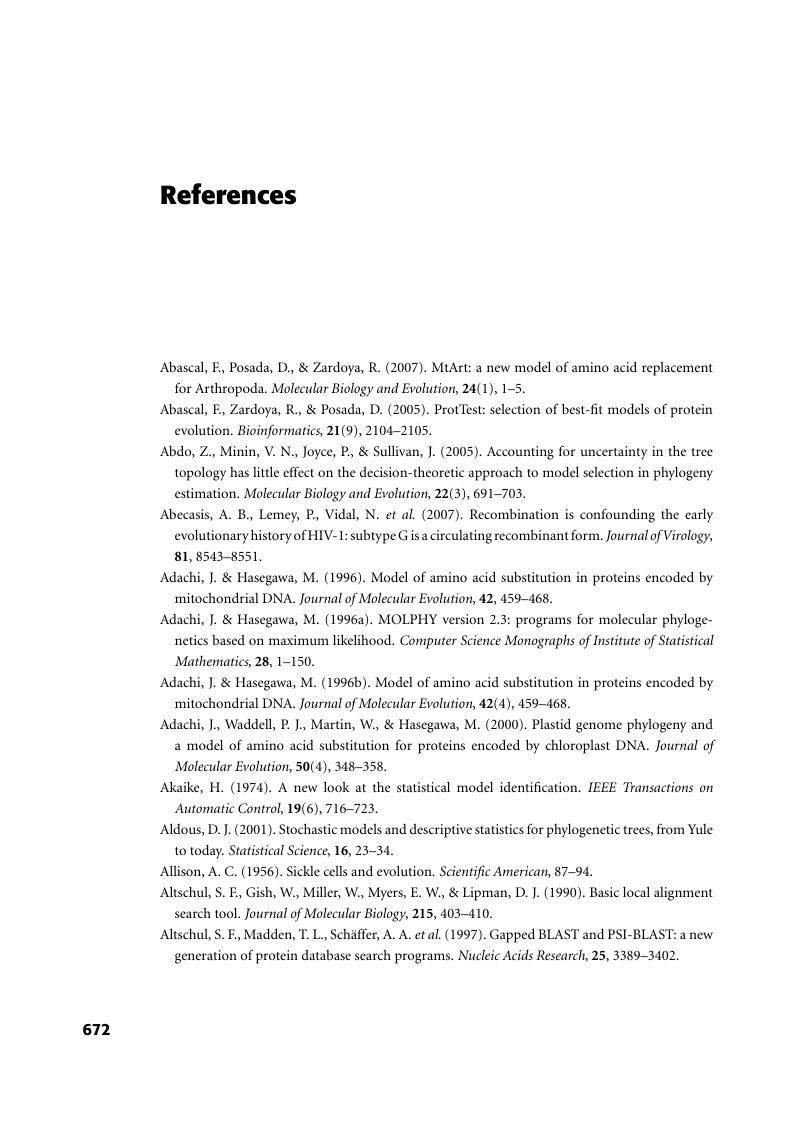
- Type
- Chapter
- Information
- The Phylogenetic HandbookA Practical Approach to Phylogenetic Analysis and Hypothesis Testing, pp. 672 - 708Publisher: Cambridge University PressPrint publication year: 2009