Book contents
- Frontmatter
- Contents
- Contributors
- Preface
- SECTION I CHARACTERIZATION OF CANCER STEM CELLS
- SECTION II THERAPEUTIC IMPLICATIONS OF CANCER STEM CELLS
- 5 Preventative and therapeutic strategies for cancer stem cells
- 6 Targeting acute myelogenous leukemia stem cells
- SECTION III TARGETING CANCER STEM CELL PATHWAYS
- Index
- Plate section
- References
6 - Targeting acute myelogenous leukemia stem cells
from SECTION II - THERAPEUTIC IMPLICATIONS OF CANCER STEM CELLS
Published online by Cambridge University Press: 15 December 2009
- Frontmatter
- Contents
- Contributors
- Preface
- SECTION I CHARACTERIZATION OF CANCER STEM CELLS
- SECTION II THERAPEUTIC IMPLICATIONS OF CANCER STEM CELLS
- 5 Preventative and therapeutic strategies for cancer stem cells
- 6 Targeting acute myelogenous leukemia stem cells
- SECTION III TARGETING CANCER STEM CELL PATHWAYS
- Index
- Plate section
- References
Summary
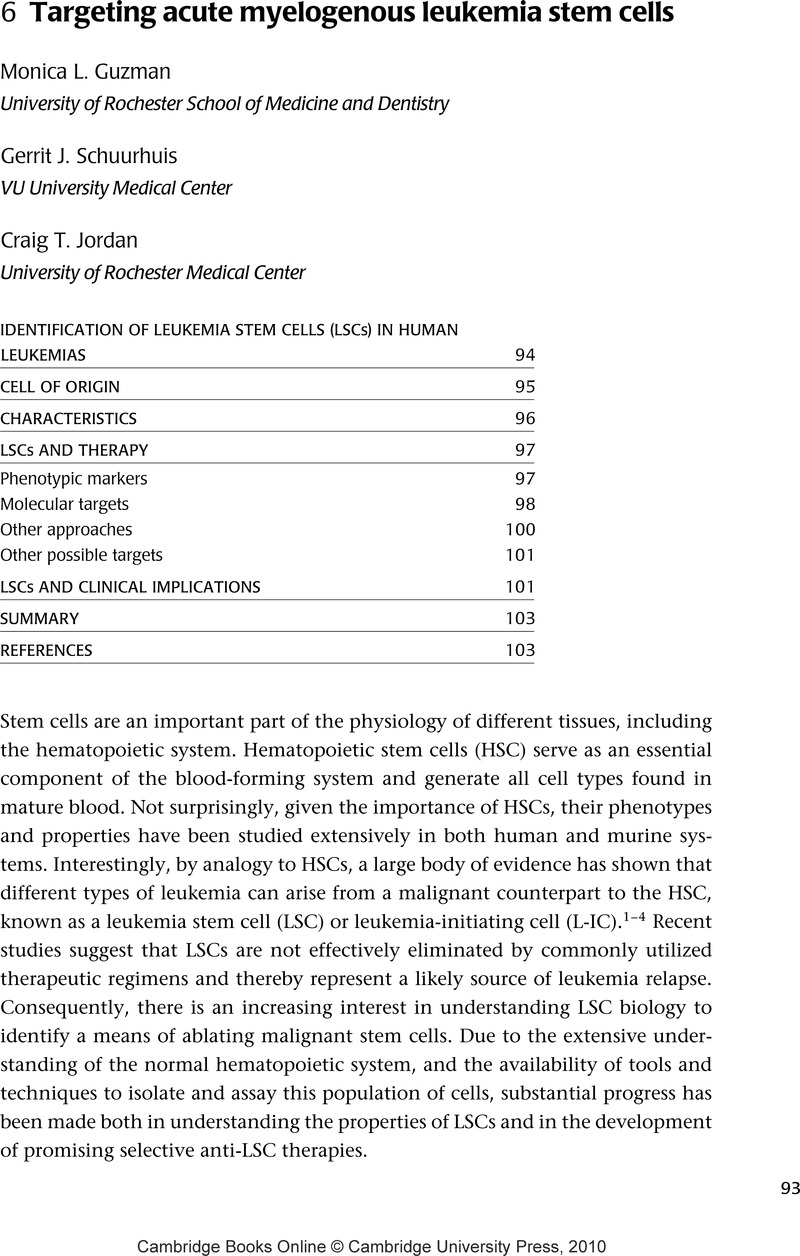
- Type
- Chapter
- Information
- Cancer Stem Cells , pp. 93 - 108Publisher: Cambridge University PressPrint publication year: 2009