Book contents
- Integrative Mechanobiology
- Integrative Mechanobiology
- Copyright page
- Contents
- Contributors
- Preface
- Part I Micro-nano techniques in cell mechanobiology
- Part II Recent progress in cell mechanobiology
- 12 Forces of nature
- 13 Mechanobiological stimulation of tissue engineered blood vessels
- 14 Bone cell mechanobiology using micro- and nano-techniques
- 15 Molecular mechanisms of cellular mechanotransduction in wound healing
- 16 Micropost arrays as a means to assess cardiac muscle cells
- 17 Micro- nanofabrication for the study of biochemical and biomechanical regulation of T cell activation
- 18 Study of tumor angiogenesis using microfluidic approaches
- 19 Neuromechanobiology of the brain
- Index
- References
16 - Micropost arrays as a means to assess cardiac muscle cells
from Part II - Recent progress in cell mechanobiology
Published online by Cambridge University Press: 05 November 2015
- Integrative Mechanobiology
- Integrative Mechanobiology
- Copyright page
- Contents
- Contributors
- Preface
- Part I Micro-nano techniques in cell mechanobiology
- Part II Recent progress in cell mechanobiology
- 12 Forces of nature
- 13 Mechanobiological stimulation of tissue engineered blood vessels
- 14 Bone cell mechanobiology using micro- and nano-techniques
- 15 Molecular mechanisms of cellular mechanotransduction in wound healing
- 16 Micropost arrays as a means to assess cardiac muscle cells
- 17 Micro- nanofabrication for the study of biochemical and biomechanical regulation of T cell activation
- 18 Study of tumor angiogenesis using microfluidic approaches
- 19 Neuromechanobiology of the brain
- Index
- References
Summary
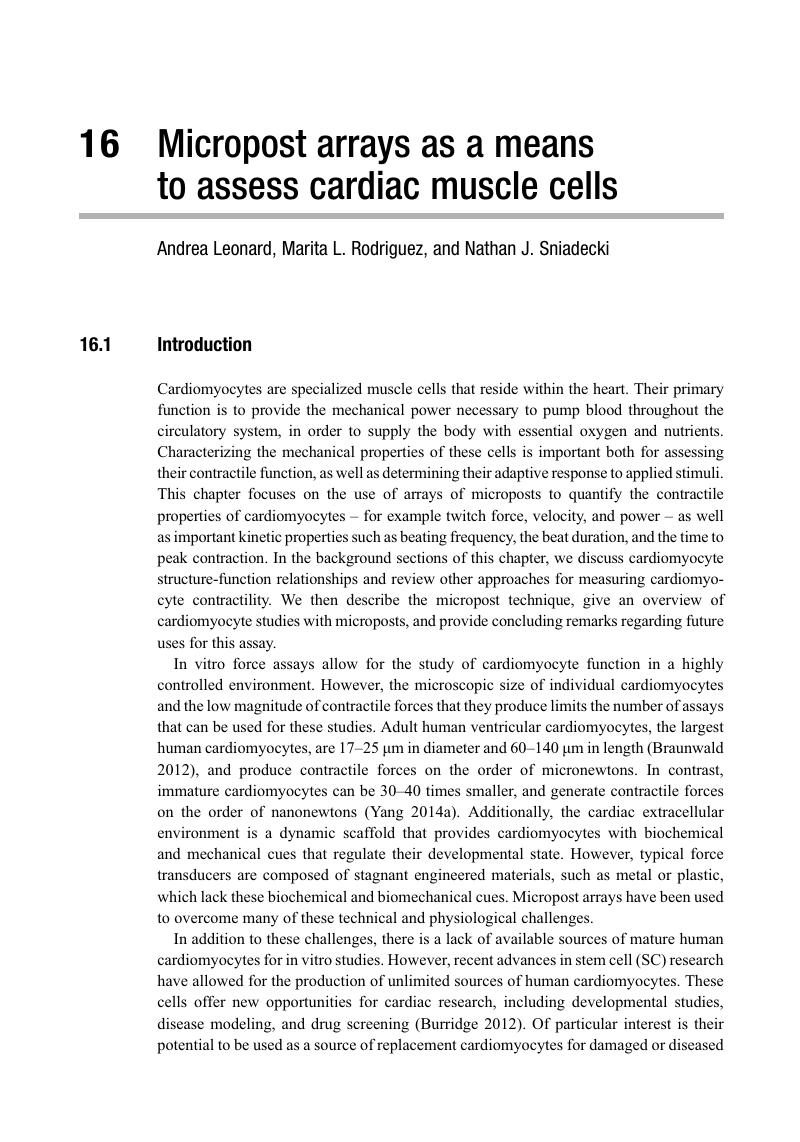
- Type
- Chapter
- Information
- Integrative MechanobiologyMicro- and Nano- Techniques in Cell Mechanobiology, pp. 295 - 315Publisher: Cambridge University PressPrint publication year: 2015