Book contents
- Frontmatter
- Contents
- Preface
- Nomenclature
- 1 Introduction
- 2 Engineering Seismology
- 3 Basics of Random Processes and Structural Dynamics
- 4 Seismic Response Spectra
- 5 Seismic Hazard Analysis
- 6 Ground Motions for Seismic Analysis
- 7 Modelling of Structures
- 8 Floor Response Spectra
- 9 Seismic Fragility Analysis
- 10 Seismic Probabilistic Safety Assessment
- Appendix A Basics of Normal and Lognormal Distributions
- Appendix B Digital Signal Processing
- Bibliography
- Index
- References
Bibliography
Published online by Cambridge University Press: 29 March 2019
- Frontmatter
- Contents
- Preface
- Nomenclature
- 1 Introduction
- 2 Engineering Seismology
- 3 Basics of Random Processes and Structural Dynamics
- 4 Seismic Response Spectra
- 5 Seismic Hazard Analysis
- 6 Ground Motions for Seismic Analysis
- 7 Modelling of Structures
- 8 Floor Response Spectra
- 9 Seismic Fragility Analysis
- 10 Seismic Probabilistic Safety Assessment
- Appendix A Basics of Normal and Lognormal Distributions
- Appendix B Digital Signal Processing
- Bibliography
- Index
- References
Summary
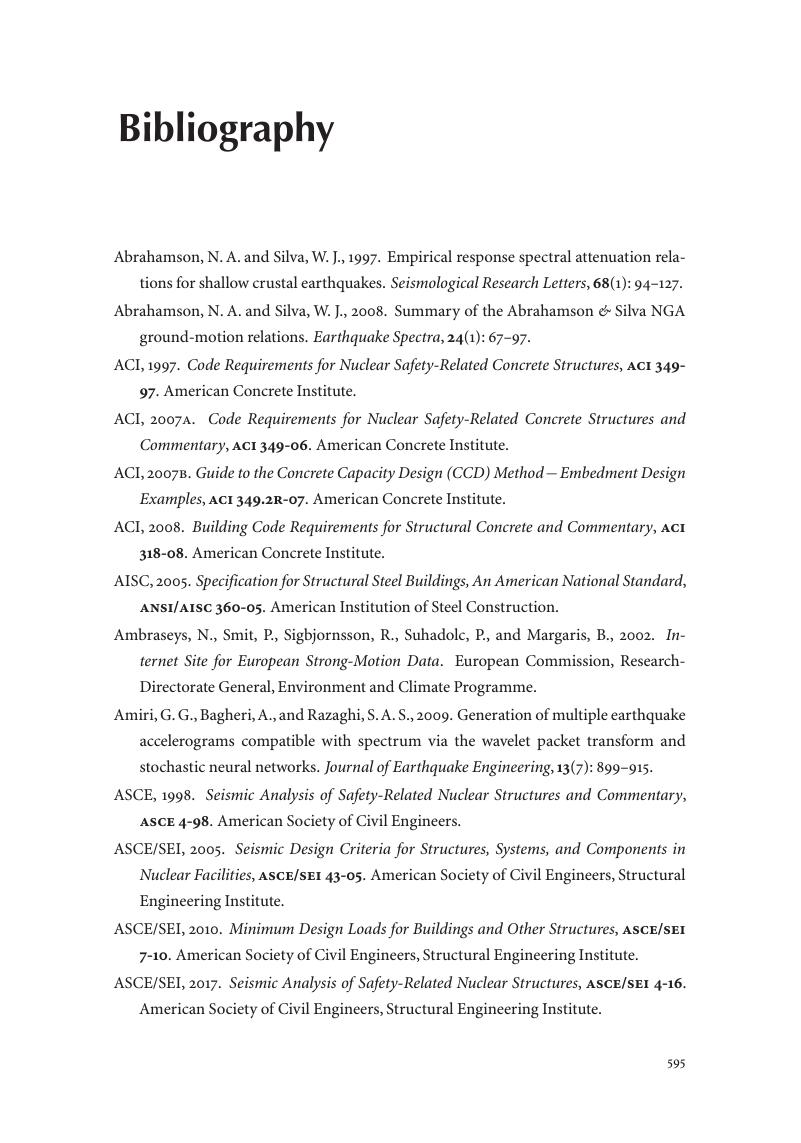
- Type
- Chapter
- Information
- Seismic Risk Analysis of Nuclear Power Plants , pp. 595 - 608Publisher: Cambridge University PressPrint publication year: 2019