Book contents
- The Global Cryosphere, Second Edition
- Reviews
- The Global Cryosphere
- Copyright page
- Contents
- Preface
- Acknowledgments
- 1 Introduction
- Part I The terrestrial cryosphere
- Part II The marine cryosphere
- Part III The cryosphere past and future
- Part IV Applications
- References
- Index
- Plate Section (PDF Only)
- References
References
Published online by Cambridge University Press: 15 April 2022
- The Global Cryosphere, Second Edition
- Reviews
- The Global Cryosphere
- Copyright page
- Contents
- Preface
- Acknowledgments
- 1 Introduction
- Part I The terrestrial cryosphere
- Part II The marine cryosphere
- Part III The cryosphere past and future
- Part IV Applications
- References
- Index
- Plate Section (PDF Only)
- References
Summary
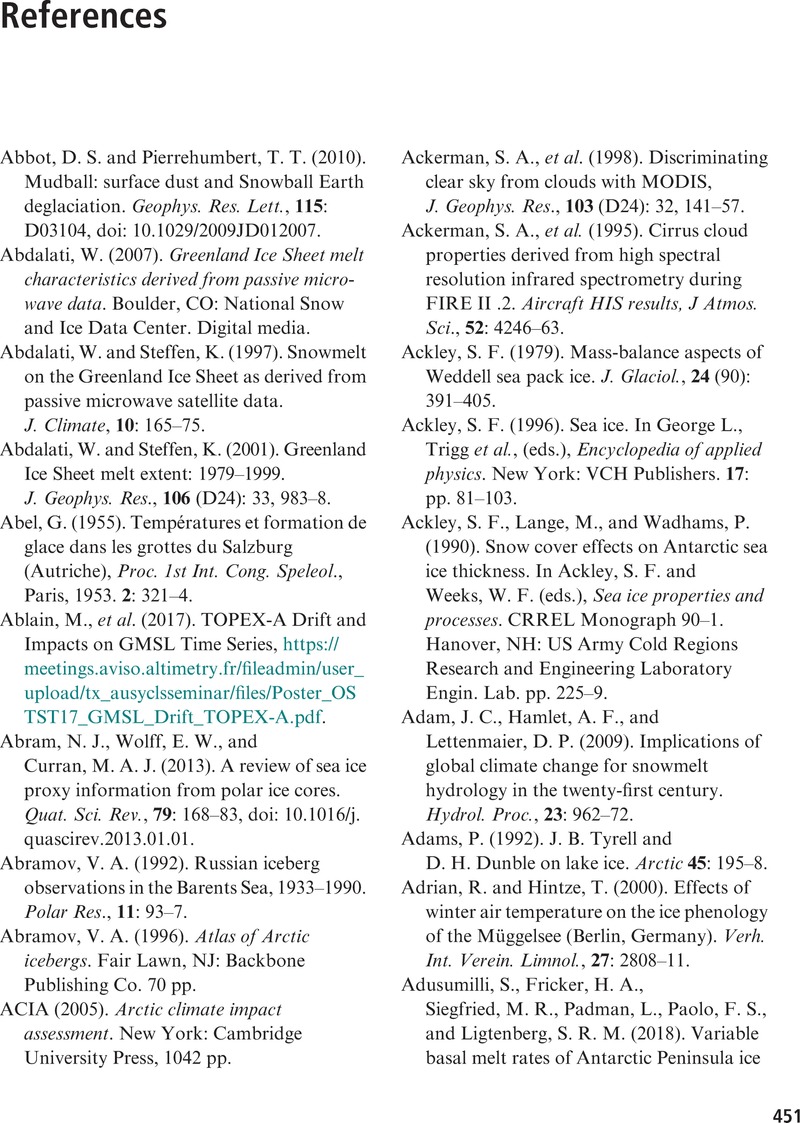
- Type
- Chapter
- Information
- The Global CryospherePast, Present, and Future, pp. 451 - 562Publisher: Cambridge University PressPrint publication year: 2022