Book contents
- Frontmatter
- Contents
- Preface
- Acknowledgements
- Part I Electromagnetic Theory in the Optical Domain
- Part II Geometrical Theory of Optical Imaging
- Part III Diffraction Theory of Optical Imaging
- Appendix A Fourier Analysis, Complex Notation and Vector Formulas
- Appendix B Phase and Group Velocity of a Wave Packet
- Appendix C The Kramers–Kronig Dispersion Relations
- Appendix D Zernike Polynomials
- Appendix E Magnetically Induced Optical Rotation (Faraday Effect)
- Appendix F Vector Point-spread Function in a Multilayer Structure
- Appendix G V. S. Ignatowsky: Diffraction by a Lens of Arbitrary Aperture
- References
- Author Index
- Subject Index
- References
References
Published online by Cambridge University Press: 22 April 2019
- Frontmatter
- Contents
- Preface
- Acknowledgements
- Part I Electromagnetic Theory in the Optical Domain
- Part II Geometrical Theory of Optical Imaging
- Part III Diffraction Theory of Optical Imaging
- Appendix A Fourier Analysis, Complex Notation and Vector Formulas
- Appendix B Phase and Group Velocity of a Wave Packet
- Appendix C The Kramers–Kronig Dispersion Relations
- Appendix D Zernike Polynomials
- Appendix E Magnetically Induced Optical Rotation (Faraday Effect)
- Appendix F Vector Point-spread Function in a Multilayer Structure
- Appendix G V. S. Ignatowsky: Diffraction by a Lens of Arbitrary Aperture
- References
- Author Index
- Subject Index
- References
Summary
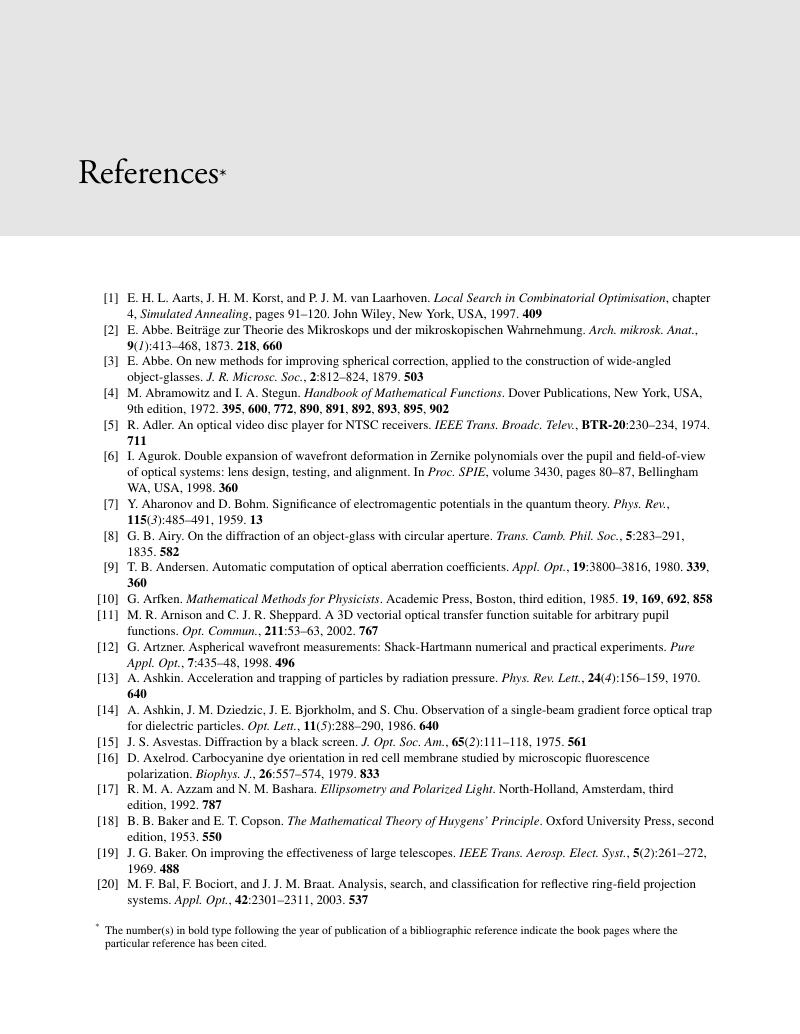
- Type
- Chapter
- Information
- Imaging Optics , pp. 945 - 958Publisher: Cambridge University PressPrint publication year: 2019