Introduction
Antarctica is a continent characterized by volcanism, which has repeatedly occurred from Palaeozoic times, at least, until present (LeMasurier & Thomson Reference LeMasurier and Thomson1990, Smellie Reference Smellie, Oliva and Ruiz-Fernández2020, Smellie et al. Reference Smellie, Panter and Geyer2021a and references therein). It was particularly voluminous and widespread in the Jurassic, comprising flood basalts and related intrusions coinciding with the break-up of Gondwana, but it also includes the products of a major long-lived (Mesozoic–Cenozoic) continental margin magmatic arc (Leat & Riley Reference Leat and Riley2021) and hosts one of the world's great intra-continental rift zones with associated alkaline magmas (the West Antarctic Rift System; Jordan et al. Reference Jordan, Riley and Siddoway2020, Wilch et al. Reference Wilch, McIntosh and Panter2021). Antarctica is still volcanically active, with at least 12 volcanoes that are classed as active or potentially so (e.g. Deception Island, Mount Erebus, Mount Melbourne, Mount Berlin; Geyer Reference Geyer2021). They include three known centres that are entirely subglacial and were detected remotely by geophysical methods (Mount Casertz, Hudson Mountains Subglacial Volcano and an unnamed centre south of Mount Waesche in central Marie Byrd Land: Blankenship et al. Reference Blankenship, Bell, Hodge, Broxena, Behrendt and Finn1993, Corr & Vaughan Reference Corr and Vaughan2008, Lough et al. Reference Lough, Wiens, Barcheck, Anandakrishnan, Aster and Blankenship2013), although many more may exist and remain to be verified (van Wyk de Vries et al. Reference Van Wyk de Vries, Bingham and Hein2018). In the 1820s, sealers made up the majority of the early explorers to the South Shetland Islands. Although exact numbers of persons are unknown, US and British sealing vessels peaked at 44 and 91 ships, respectively, in the summers of 1820–1821 and 1821–1822 (Headland Reference Headland2009, p. 123). It was they who reported volcanic activity from Bridgeman Island within 2 years of Antarctica being discovered (Williams Reference Williams2021). Thus, Bridgeman Island lays claim to being the first Antarctic volcano to be observed and the first seen in an eruptive state. In January 1841, 20 years later, Mount Erebus volcano (Ross Sea region) was erupting when it was discovered (Ross Reference Ross1847). Deception Island (South Shetland Islands), recognized as an old volcano from the 1820s, was seen erupting for the first time in February 1842 by sealing captain William H. Smiley of the Ohio (Wilkes Reference Wilkes1845).
Bridgeman Island is an enigma. Although formed entirely of volcanic rocks, the youngest of which have a K-Ar isotopic age of just 63 ka (with high errors (± 25 ka); González-Ferrán & Katsui Reference González-Ferrán and Katsui1970, Keller et al. Reference Keller, Fisk, White and Birkenmajer1992), there are no primary volcanic landforms. However, Matthies et al. (Reference Matthies, Storzer and Troll1988) identified a marine tephra layer in a sediment core from Bransfield Strait, which they matched compositionally with Bridgeman Island, although the analyses were not published. The age, location and depth of the tephra layer are unknown but, if verified, the presence of the tephra layer would confirm Holocene activity sourced on Bridgeman Island. The historical reports of explosive volcanic activity have been disputed principally owing to the absence of any primary volcanic landforms associated with such a young volcanic centre. Moreover, a detailed sketch of Bridgeman Island made in 1838 suggested that the island had not changed its morphology significantly since its discovery (Dumont D'Urville Reference Dumont D'Urville1842, Grange Reference Grange1848). Thus, despite maps such as that by Weddell (Reference Weddell1825), which clearly shows both Bridgeman Island and Penguin Island (called Georges Island on Weddell's map; see also Sherratt Reference Sherratt1821), a consensus arose that the supposed eruption(s) of Bridgeman Island actually took place on Penguin Island. The latter is a small volcano ~1.5 km in diameter with well-preserved volcanic features (prominent scoria cone and a maar) situated 60 km west of Bridgeman Island and with probable historical eruptive episodes (González-Ferrán & Katsui Reference González-Ferrán and Katsui1970, Birkenmajer Reference Birkenmajer1979, Smellie Reference Smellie, LeMasurier and Thomson1990, Global Volcanism Program 2013). However, a major reappraisal of the numerous contemporary reports by Williams (Reference Williams2021) has convincingly demonstrated that the events attributed to Bridgeman Island could not have been misplaced and should not be reassigned to Penguin Island. Verification of the reported eruption remains problematical, however, because of the lack of any physical evidence.
In this paper, we concur with the view that Bridgeman Island did erupt in the early 19th century. We review the evidence for the eruption provided by the historical reports and reinterpret it in terms of the probable eruptive style and sequence of events. We also show how the volcano might have looked during its eruptive period and interpret it as a short-lived hydrovolcanic Surtseyan eruption that has left no visible geological record.
Geological setting
Bridgeman Island is situated ~45 km east of King George Island (South Shetland Islands), in the centre of Bransfield Strait (Fig. 1). Bransfield Strait is a small marginal basin representing the last relict of subduction-related processes affecting the Pacific margin of Antarctica. Subduction at the Pacific trench ceased progressively in a northerly direction as a result of collisions of offset segments of an oceanic spreading centre (Barker Reference Barker1982, Larter et al. Reference Larter, Cunningham and Barker2002). However, at the South Shetland Islands, collision did not occur and subduction continued, although at a very slow rate, followed by the opening up of a small rift basin between the islands and northern Antarctic Peninsula, probably in the last 4 Myr (Larter Reference Larter1991, Maldonado et al. Reference Maldonado, Larter and Aldaya1994). The precise origin of the basin (also called the Bransfield Rift) is still debated, with two hypotheses predominant. In the first, the basin is linked to slab roll-back following the cessation of spreading in Drake Passage and it is regarded as at the rift:drift transition (Barker Reference Barker1982, Barker & Austin Reference Barker and Austin1994, Reference Barker and Austin1998). In the second hypothesis, basin opening is attributed to sinistral shear between the Scotia and Antarctic plates, creating compression on the Shackleton fracture zone, locking the South Shetland trench and causing oblique extension (Klepeis & Lawver Reference Klepeis and Lawver1996, González-Casado et al. Reference González-Casado, Giner-Robles and López-Martínez2000, Fretzdorff et al. Reference Fretzdorff, Worthington, Haase, Hekinian, Franz, Keller and Stoffers2004). North-west to south-east extension occurs across the region, consistent with both hypotheses (Pelayo & Wiens Reference Pelayo and Wiens1989, González-Casado et al. Reference González-Casado, Giner-Robles and López-Martínez2000), but the presence of continuing slow subduction is inconsistent with a locked South Shetland trench (Robertson Maurice et al. Reference Robertson Maurice, Wiens, Shore, Vera and Dorman2003).

Fig. 1. Map of the Bransfield Strait region showing the location of Bridgeman Island and distribution of submarine volcanic edifices and volcanic islands (based on Smellie (Reference Smellie2021b), modified). The bold black line surrounding Bridgeman Island represents the inferred base of the volcano that has grown on top of Bridgeman Rise.
Arc volcanism in the South Shetland Islands dates back to the earliest Cretaceous (Leat & Riley Reference Leat and Riley2021). It evolved as a series of relatively narrow north-easterly trending volcanic ‘belts’ that migrated progressively in a south-easterly direction, possibly in response to forearc erosion due to an oblique plate convergence vector (Smellie Reference Smellie2021a). The youngest volcanic rocks are Oligocene–early Miocene in age and are confined to the south coast of King George Island (Smellie et al. Reference Smellie, McIntosh, Whittle, Troedson and Hunt2021b). However, the Bransfield Rift, which also has a north-easterly elongation, is a major locus for Quaternary volcanism, and some of that volcanism might be a continuation of the south-easterly migratory trend. The rift is characterized by thinned crust with high heat flow, seismicity, hydrothermalism and active volcanism (Lawver et al. Reference Lawver, Ghidella, von Herzen and Keller1996). It has been divided into three sub-basins, designated western, central and eastern. They are probably separated by major crustal discontinuities and may be linked in some fashion to oceanic transform faults offshore, to which the inferred crustal discontinuities are parallel (Birkenmajer Reference Birkenmajer2001, fig. 2). Bridgeman Island is situated at the junction between the central and eastern basins (Fig. 1).
Bransfield Rift contains numerous small submarine seamounts and ridges and islands that include Bridgeman Island and Deception Island, one of the most active Antarctic volcanoes (Fig. 1; Gràcia et al. Reference Gràcia, Canals, Farràn, Prieto and Sorribas1996, Geyer et al. Reference Geyer, Pedrazzi, Almendros, Berrocoso, López-Martínez and Maestro2021). The volcanism is mainly basaltic, characterized by distinctive Na-rich compositions, but it includes rare rhyolites (Keller et al. Reference Keller, Fisk, White and Birkenmajer1992, Reference Keller, Fisk, Smellie, Strelin and Lawver2002, Fretzdorff et al. Reference Fretzdorff, Worthington, Haase, Hekinian, Franz, Keller and Stoffers2004). The basalts are compositionally relatively like mid-ocean ridge basalts (MORBs) in the south-west, changing to more arc-like in the north-east, but the variations are not systematic. Bridgeman and Deception islands have been regarded as remnants of the South Shetland arc volcanism (Barker et al. Reference Barker, Christeson, Austin and Dalziel2003), but the most arc-like examples occur in a cluster of seamounts in the Eastern Bransfield Basin near Gibbs Rise (Fretzdorff et al. Reference Fretzdorff, Worthington, Haase, Hekinian, Franz, Keller and Stoffers2004). Although Deception Island is the largest single volcano in the Bransfield Rift, Bridgeman Island is situated on top of a substantial submarine massif known as Bridgeman Rise measuring 10 km × 15 km in basal diameter and extending to a depth of ~1200 m (Fig. 1). It is volumetrically the second-largest volcanic construct in the rift but is slightly taller than that forming Deception Island, rising to 1050 m from its base compared with 1000 m for Deception Island (Gràcia et al. Reference Gràcia, Canals, Farràn, Prieto and Sorribas1996).
Methods
This study is mainly based on a volcanological reinterpretation of the historical reports of volcanic activity on Bridgeman Island. It is supplemented by information gained during a short visit to the summit region of Bridgeman Island by SK on 22 January 2008 and an examination of numerous hand-held oblique aerial photographs of the island taken during that visit, which were used to interpret the geology of the island and prepare a new geological map.
Geology of Bridgeman Island
Bridgeman Island measures 900 m × 600 m and rises to 240 m. It is surrounded on all sides by inaccessible vertical cliffs that rise almost to the summit. The island has a smooth undulating upper surface, and it was often described as ‘conical’ or like ‘a sugar loaf’ in the early historical reports (Williams Reference Williams2021). The French expedition of Dumont D'Urville observed Bridgeman Island closely on 26 February 1838 over a period of 4 h. Two small rowing boats, launched from the ships Astrolabe and Zélée, came within metres of the island. They circled Bridgeman Island but were unable to land due to the swell. However, its geology was described in outline, including the presence of several active fumaroles (Dumont D'Urville Reference Dumont D'Urville1842). A sketch of the island was also made (Grange Reference Grange1848). The only other description based on a geological study on the island itself is by González-Ferrán & Katsui (Reference González-Ferrán and Katsui1970), and all other published descriptions have simply reworked the information provided by that study. Two geological units have been identified, with subalkaline basalt and basaltic andesite compositions (Figs 2 & 3; Weaver et al. Reference Weaver, Saunders, Pankhurst and Tarney1979, Kraus et al. Reference Kraus, Kurbatov and Yates2013). The lower unit comprises crudely bedded agglomerate, scoria lapillistone and tuff, mainly bright red (oxidized) but varying to grey, and thin (1–7 m) lavas, many of which are probably clastogenic (Fig. 4). The lavas are mostly developed at the south-east corner of the island, but much thicker lavas (individually ~30 m?) probably form the lower half of the western cliffs. The strata dip in opposing directions in the eastern half of the island, indicating a former crater probably situated to the west or south-west. The lower unit is cut by an uneven unconformity that dips broadly to the north or north-north-east and extends down to sea level (Fig. 3). The unconformity is overlain by an upper unit composed predominantly of thick (25–30 m) lavas (also mainly clastogenic), which are thickest in the western cliffs and become thinner to the east, where they are interbedded with intensely red-coloured scoria lapillistones and minor tuff (Fig. 4). Dips in the upper unit are essentially quaquaversal and suggest a former vent to the west of the island. The surface of the upper unit is relatively smooth and curviplanar. It appears to be largely unmodified by erosion. Both geological units are remnants of scoria cones. At least some of the thick lavas exposed in the western cliffs may have ponded within lava lakes. Only the upper unit has been dated, yielding a radioisotopic age of 63 ± 25 ka (Keller et al. Reference Keller, Fisk, White and Birkenmajer1992).

Fig. 2. Geological sketch map of Bridgeman Island, based on González-Ferrán & Katsui (Reference González-Ferrán and Katsui1970) and new photographic observations. Map drawn onto FIDASE aerial photograph X26FID0041051A (available at http://earthexplorer.usgs.gov/).

Fig. 3. Views of Bridgeman Island (photographs taken in 1975) with the geology draped on. The insets on each image show the viewing direction (arrowhead). a. View looking west. b. View looking south-west. c. View looking east-south-east. d. View looking north-north-west. Note the smooth uneroded surface of the summit of the island, which mimics the orientation of underlying strata.

Fig. 4. View of Bridgeman Island looking to the south-west. Note the intense red colouration that pervades the entire sequence, attributed to oxidation of Strombolian scoria and agglutinate deposits, and the variable dip directions in the lower unit. The clastogenic nature of most of the lavas is clearly evident in the upper unit. Photograph by Jeronimo López-Martínez.
Review and volcanological reinterpretation of the historical accounts
Williams (Reference Williams2021) summarized the descriptions included in all relevant accounts of Bridgeman Island following its discovery in January 1820 until the early 20th century (Table I). Of the 12 accounts included by Williams (Reference Williams2021), only two provide meaningful details that can be interpreted volcanologically, with minimal ambiguity. The most informative is by Captain George Powell from 18 December 1821 (Powell Reference Powell1822a,Reference Powellb), which enables us to identify the type of eruption that took place. Captain Powell witnessed the island initially from a position 9 miles (14 km) to the north-east. In his description, he mentions ‘smoke’ being emitted from five craters but, when viewed closer in (~1.5 miles distant (2.4 km)), he says that it was ‘but one crater, of immense width, from which smoke issued in dark volumes; … the smoke branched off into the different columns of the rocks'. He further indicated that ‘the crater is situated on the west-side of the island, and is about 80 or 90 feet [25–27 m] from the surface of the sea'. Finally, he provided a sketch of the island that is approximately rectangular in plan view, pointed at the south-west end, and shows a prominent crater offset to the south-west (Fig. 5). Interestingly, the crude sketch of Bridgeman Island by Sherratt (Reference Sherratt1821) is broadly similar in shape to that provided by Powell. It also apparently shows higher ground at the eastern end of the island but no crater. By contrast, Powell's description and sketch indicate a large crater with a relatively low rim < 30 m above sea level, observations that most resemble a tuff ring landform rather than a tuff cone and suggesting an eruption in very shallow water (cf. Wohletz & Sheridan Reference Wohletz and Sheridan1983, Németh & Kereszturi Reference Németh and Kereszturi2015, Németh & Kósik Reference Németh and Kósik2020). Information is sparse regarding water depths on the marine platform surrounding Bridgeman Island. They are mainly ~30–60 m, although greater in the west (to 180 m) and north (> 200 m) within a few kilometres (UK Admiralty Chart 1949). Such shallow depths, with the erupting vent flooded by seawater, would normally promote the construction of a tuff cone rather than a tuff ring unless the edifice was built on an unusually shallow part of the platform, but the report and sketch by Powell most closely fit with a tuff ring. The description of smoke apparently emitted from five craters, yet only a single crater seen when Powell was close enough to be certain, suggests an eruption that shut off suddenly after repeated explosive events. After each shut-off, eruption plumes frequently drift downwind as detached vertical columns, giving the spurious impression of eruptions from multiple vents (Fig. 6). Of greatest potential importance, however, is Powell's description of the columns themselves, with the ‘smoke’ branching off in different directions. This description is extraordinarily similar to modern descriptions of eruptive jets sourced in individual hydrovolcanic explosions and known as cypressoid (also cocks-tail or tephra finger) jetting activity (Thorarinsson et al. Reference Thorarinsson, Einarsson, Sigvaldason and Elisson1964, Moore Reference Moore1985). The ‘dark volumes’ undoubtedly refer to the grey coloration imparted by ash entrained in an eruption column or jets (Fig. 6). Thus, from Powell's account we can surmise that an eruption was taking place from a small volcano with a low (30 m) broad crater, situated in the sea (therefore hydrovolcanic) and characterized by cypressoid jetting activity that repeatedly shut off and resumed.

Fig. 5. Expanded view of part of the chart prepared by Powell (Reference Powell1822a) showing his sketch of Bridgeman Island. Note how close Powell sailed to the island, which would have given him an excellent view of its appearance. Note also the elongate, crudely rectangular shape of the island with its pointed south-western termination and a broad crater offset to the south-west.

Fig. 6. Aerial view of a vertical eruption column that has detached and drifted downwind after a sudden shutdown of explosive hydrovolcanic activity at its vent (vent obscured by low cloud). The column is grey due to the presence of fine ash. Continuing vigorous fumarolic activity at the erupting vent is revealed by the off-white steam plume (poor or free of ash) seen at the right drifting downwind at low elevation. Eruption of Mount Belinda, Montagu Island, South Sandwich Islands, March 2006 (photograph by J.L. Smellie; see Patrick et al. Reference Patrick, Smellie, Harris, Wright, Dean and Izbekov2005 for eruption details).
Table I. History of relevant observations of Bridgeman Island, arranged chronologically, and interpretation.


Fig. 7. Comparison of views of the south end of Bridgeman Island showing topographical changes since 1838. Substantial similarities exist for features shown within boxes 1 and 2 in both figures, whereas comparison of box 3 shows significant changes probably caused by major erosion or collapse events. The 1838 headland (box 3 shown in a.; sketched by Grange Reference Grange1848) was accessible and therefore a popular location for nesting penguins, noted as early as 1821 by Powell (Reference Powell1822b). In a., box 4 apparently shows land that is clearly absent in box 4 shown in b. The land may be a remnant of the 1821 new island still present in 1838, when Grange (Reference Grange1848) drew the sketch.
The second most useful account was by sealer Nathaniel Ames, who observed Bridgeman Island months before Powell, between December 1820 and March 1821, describing it as an island ‘burning fiercely and sending forth a thick sulphurous smoke’ (Ames Reference Ames1830). He further described ‘a mere rock of conical form and no great size’ that ‘broke out with considerable violence'. He also saw a ‘light by night', together with a crater ‘burning fiercely and sending forth a thick sulphurous smoke', as well as ‘numbers of dead penguins … found floating about’ and supposedly ‘overcome by the difference in temperature’ (Ames Reference Ames1830). Ames’ report of a crater is the first description of a volcanic landform on the island. The observation of dead penguins indicates that he managed to get close to the volcano, and he records that he visited it in a rowing boat. Although he noted the presence of a crater, he provided no sketch. Taken together with Powell's account, the observations are mutually supporting and strongly suggest that Ames also observed a crater with a plume. Like Williams (Reference Williams2021), we suggest that the ‘light by night’ was a reference to incandescence from a volcanic plume. It is well known that hydrovolcanic eruption columns, particularly those formed during continuous uprush activity, are incandescent under low-light conditions (Thorarinsson et al. Reference Thorarinsson, Einarsson, Sigvaldason and Elisson1964, Moore Reference Moore1985, Cole et al. Reference Cole, Guest, Duncan and Pacheco2001). The mention of ‘light by night’ implies that Ames’ observations were probably made during February or March 1821, when nights would have become gloomy then dark, respectively, at the high southern latitude. They clearly predate the observations by Powell, made ~9 months later, on 18 December 1821. The penguin mortality is unlikely to have been caused by temperature differences. Penguins are hardy animals quite capable of tolerating wide temperature fluctuations (P. Trathan, British Antarctic Survey, personal communication 2019). They are also very mobile in water and able to swim rapidly away from any ‘hotspots’ before they approach mortality. It is more plausible that the deaths were caused by sudden emissions of superheated gases or else the percussive effects of volcanic detonations, which would have been particularly effective had the crater been breached and the penguins were swimming inside during a lull in activity. If cypressoid jetting activity was occurring, observations of modern eruptions suggest that the crater was probably breached at times (Thorarinsson et al. Reference Thorarinsson, Einarsson, Sigvaldason and Elisson1964). Ames observed the volcano when the construct was probably much less well developed than when Powell saw it many months later. At the time it may have been a simple ring of tephra with a low crater rim exposed above sea level. Constructed from loose pyroclastic material, breaching by the sea would have been comparatively easy.
Most of the other historical observations are less revealing, varying from simply calling the island ‘a burning mount’ or mentioning ‘smoke’ issuing from it (Table I). The latter is a common epithet, but it is particularly difficult to link unambiguously with an ongoing eruption without further corroboration as meteorological effects linked to insolation and topography, or even a strong wind, can generate apparent small plumes (e.g. report by Jean-Baptiste Charcot (Reference Charcot and Walsh1911), observation made in 1909; Table I). However, given the much less ambiguous reports by Powell and Ames, at least some of the contemporaneous reports were almost certainly describing active volcanism. Some also mention ‘smoke’ with a sulphurous smell, which Williams (Reference Williams2021) not unreasonably suggested was proof of fumarolic activity. Reports that lack mention of an associated smell are more ambiguous. James Weddell also noted ‘smoke issuing through fissures in the rocks, and apparently with much force’ (emphasis added) in late 1821 (Table I). Given that the date is after the observations of Ames and at approximately the same time as those made by Powell, a fumarolic origin is certain, but the locus of the activity appears to be on the conical island (i.e. on the rock currently known as Bridgeman Island) rather than on a low-lying ‘new’ volcano, as postulated here.
When did the eruption commence? The first mention of Bridgeman Island was by Edward Bransfield, RN, on 21 January 1820 (Table I; Campbell Reference Campbell2000). The island was noted while his ship rounded Cape Melville, which forms the easternmost promontory of King George Island ~45 km west of Bridgeman Island (Fig. 1). No comment on any volcanic activity was made on that voyage of discovery, which might be taken as evidence that the volcano was not active at the time. However, because of the curvature of the Earth, an object just 30 m high will be invisible from a ship at distances exceeding ~25–30 km. Moreover, only the crater rim reached that height, and it might also have been lower at that stage in its construction; most of the volcano was considerably lower, so it would have been very easy to miss unless it happened fortuitously to be in eruption when viewed. It may be significant that Bridgeman Island was not mentioned at all when Bransfield again passed between the island and Cape Melville on 2 February 1820. However, the weather was terrible at that time ('fresh gales with hazy weather and a very heavy sea'). Bransfield was unaware that the island was a volcano and obviously saw no eruption. This suggests that the volcano was either not present, it was undergoing a pause in activity or it was not visible because of local weather conditions or the distance from the ship at the time. Approximately 10 months later, between 25 December 1820 and January 1821, Richard Sherratt drew a ‘burning mount’ on his new map of the area (Table I; Sherratt Reference Sherratt1821). Sherratt was shipwrecked and marooned at Cape Melville but rescued within ~2 weeks (Stackpole Reference Stackpole1935). Bridgeman Island was within his line of sight (although too far to see any crater 30 m high), and his observation suggests that eruptive activity may have already been underway when his party was castaway. January 1821 is taken as an approximate start date for the episode, as also supported by the reports of two other sealers (Mackay Reference Mackay1821, Ames Reference Ames1830).
When did the eruption finish? This is harder to define. The observations of Weddell late in 1821 quoted above (Table I) unequivocally refer to vigorous fumarolic activity (Williams Reference Williams2021). Given that Weddell passed within 200 m of Bridgeman Island, he might have seen a tuff ring on its west side. Either it had disappeared (removed by marine erosion; see later) or else his vantage point prevented him from getting a clear view west of the island. The evidence is equivocal, but the island and its environs were clearly affected by a shallow magma chamber capable of driving vigorous fumarolic activity, although apparently no eruptions. The next observations did not take place until 26 February 1838, when Grange (with Commander Dumont D'Urville; Table I) noted ‘thick columns of smoke'. Grange also confirmed that ‘there is no crater', although the ‘vapours … had an unbearable pungent odour'. Like the report by Weddell, it seems that explosive activity had ceased and the new volcanic edifice, or at least the crater, had vanished. Grange clearly came within metres of the island to make his detailed sketch. The sketch is reproduced by Williams (Reference Williams2021), who assumed that it represented a view of the north side, looking south. However, a closer comparison with modern photographs suggests that the view is much more likely to be the south side of the island, looking north (Fig. 7). It is mentioned because, although other authors have suggested that Bridgeman Island has changed very little since the mid-19th century, a detailed comparison of the sketch with a modern photograph (Fig. 7) indicates that the south-east point of the island has been considerably modified. Moreover, from that viewpoint, the new volcanic centre ought to be clearly visible, if it still existed. Interestingly, the sketch by Grange shows what appears to be low-lying land in the background at left of the sketch (box 4 in Fig. 7a). It may be a relict of the 1821 tuff ring as no land is present at that locality now. Despite the intervening 17 years, a shallow magma chamber was clearly still driving conspicuous fumarolic activity located on the west side of Bridgeman Island itself. Fumaroles with ‘a strong sulphureous [sic] smell’ were also present on 2 March 1829 (Charles Wilkes’ observation; Table I) and probably also on 25 December 1853 (Cyrene Clarke; Clarke Reference Clarke1854; Table I). The report by Thomas Lynch and Andrew Eldred on January 1880 of a live seal on Bridgeman Island with its fur burnt off on one side (Table I; Balch Reference Balch1904) may be apocryphal and therefore untrustworthy (Ferguson Reference Ferguson1921, Smellie, cited by Williams Reference Williams2021), but they also noted that the island ‘was then smoking'. However, by 24 December 1909, Jean-Baptiste Charcot searched for but found no traces of any volcanic activity on Bridgeman Island, whether explosive or fumarolic (Table I). Thus, the eruptive period (sensu lato) probably ended after 25 December 1853 or possibly after January 1880 but before 24 December 1909 (Charcot Reference Charcot and Walsh1911).
Volcanic evolution of Bridgeman Island
Bridgeman Island is constructed from the products of two important eruptive events. The predominance of oxidized scoria deposits suggests that the activity was mainly Strombolian, but the presence of numerous clastogenic lavas indicate probable lava fountaining and that the activity was Hawaiian at times (Sumner Reference Sumner1998). The younger event took place ~63 Kyr ago, during the latter part of the last glacial period (Marine Isotope Stage (MIS) 4), but the older event is undated. A period of strong subaerial or conceivably glacial erosion intervened between the two events. However, the uneroded pristine state of the surface of the younger deposits indicates that the island has never been overridden by wet-based ice in the last 63 Kyr. Bridgeman Island is situated near the centre of Bransfield Strait and was constructed on the summit of a large, submerged massif (Bridgeman Rise). It therefore represents the products of a shoaling and emerging volcano of Surtseyan type. Surtseyan eruptions commence as pillow lava effusion (in water depths ≥ ~200 m), followed upward by violent hydrovolcanic explosivity dominated by molten fuel-coolant interactions. Ultimately, when the volcano becomes emergent, the vent dries out and interaction with seawater is prevented. Thus, eruptions ultimately become fully subaerial (i.e. Strombolian or Hawaiian). By contrast with this idealized evolution of a Surtseyan volcanic centre, the volcanic products exposed on Bridgeman Island today were created during two major ‘dry’ subaerial eruptions. They lack any evidence for interaction with water (or ice), yet they crop out down to, and presumably below, current sea level. This indicates that Bridgeman Island has either subsided by an unknown amount since the eruptions took place or sea level has increased. If the eruptions occurred during glacial periods (demonstrable for the upper sequence, at least, with an age that falls within the last glacial period), sea level would have been lower and the surface on which the Strombolian activity occurred would probably have been above sea level. Moreover, with a probable low elevation and a location far out to sea, it is improbable that much snow or ice covered the exposed pre-eruption surface, so the eruption would not have been significantly affected by its presence. There is no evidence of further eruptions until January 1821 (Table I; Sherratt Reference Sherratt1821, Ames Reference Ames1830). The survival of Bridgeman Island is probably due mainly to the presence and protective effects of a resistant core of thick lavas that are now exposed on the west side of the island (Fig. 2), which is the dominant weather side.
From our analysis of the historical reports, it is now possible to describe the sequence of events that probably characterized an eruption that formed a new island (Fig. 8). The eruption probably began in January 1821. The activity was initially explosive and constructed a crater (Ames Reference Ames1830). Because the vent was located in the sea to the west of Bridgeman Island, it was hydrovolcanic, creating a low tuff ring that ultimately reached ~30 m in summit elevation (Powell Reference Powell1822a,Reference Powellb). Because the crater height is an eighth of the height of Bridgeman Island, the new island would not be visible from the east, or from any direction if viewed from a location farther than ~25–30 km away. By comparison with modern examples (e.g. Thorarinsson et al. Reference Thorarinsson, Einarsson, Sigvaldason and Elisson1964, Machado et al. Reference Machado, Parsons, Richards and Mulford1962, Moore Reference Moore1985, Cole et al. Reference Cole, Guest, Duncan and Pacheco2001), the explosive activity probably included cypressoid jetting during periods when the crater was breached by the sea. Surtseyan edifices commonly have half-moon craters that facilitate seawater access and promote water-induced eruptions (Thorarinsson et al. Reference Thorarinsson, Einarsson, Sigvaldason and Elisson1964, Kokelaar Reference Kokelaar1983, Moore Reference Moore1985, Mueller et al. Reference Mueller, Garde and Stendal2000, Németh et al. Reference Németh, Cronin, Charley, Harrison and Garae2006). This would also have permitted access by penguins during lulls in activity, followed by the mass mortality of those subsequently caught inside or close to the crater when explosivity resumed. Continuous-uprush activity probably occurred when the crater ring was complete and ingress by the sea was restricted. Small-volume eruptions of Surtseyan type, with a low Volcanic Explosivity Index (see Newhall & Self Reference Newhall and Self1982 for a definition), are associated with relatively low eruptive columns mainly < 10 km (e.g. Thorarinsson et al. Reference Thorarinsson, Einarsson, Sigvaldason and Elisson1964, Sparks Reference Sparks1986, Cole et al. Reference Cole, Guest, Duncan and Pacheco2001, Vaughan & Webley Reference Vaughan and Webley2010), although under special circumstances (e.g. caldera collapse) they may exceptionally reach much greater heights (> 20 km; Németh Reference Németh2022). By contrast, the dense columns characteristic of continuous-uprush events (and many cocks-tail jets) are typically much lower (≤ ~1 km). The relatively high mass discharge characteristic of continuous-uprush activity would be incandescent at night. An eruption column a few kilometres high, even if it was incandescent only in its basal 500 m, would be easily visible to Sherratt from his location just 45 km west of Bridgeman Island. Tephra produced by the eruption would be deposited as lapilli and ash both by fall and (mostly) from dilute pyroclastic density currents (e.g. Wohletz & Sheridan Reference Wohletz and Sheridan1983, White Reference White1996, Cole et al. Reference Cole, Guest, Duncan and Pacheco2001, Németh et al. Reference Németh, Cronin, Charley, Harrison and Garae2006). The deposits would be dominated by sideromelane-rich lapilli and ash initially potentially quite rich in fragments of the local basement torn from the vent throat during shallow molten fuel-coolant interaction explosions. Because of the shallow water, the new island probably would have rapidly expanded until it joined up with Bridgeman Island a short distance to the east, but, as activity waned, erosion by the sea would have rapidly modified the island outline. This may explain the unusual (for a volcano) approximately rectangular shape sketched by Powell in December 1821. Draping Powell's sketch onto a modern map of Bridgeman Island suggests that the crater ('Powell's crater’ in Fig. 8) was ~500–600 m in diameter and the new island was > 2 km in length. This yields an approximate circumference of 5 km (~3.1 miles) for the reconstructed island with its wave-modified coastline (Fig. 8), which is not greatly different from the 4 mile circumference estimated by Powell in late 1821.
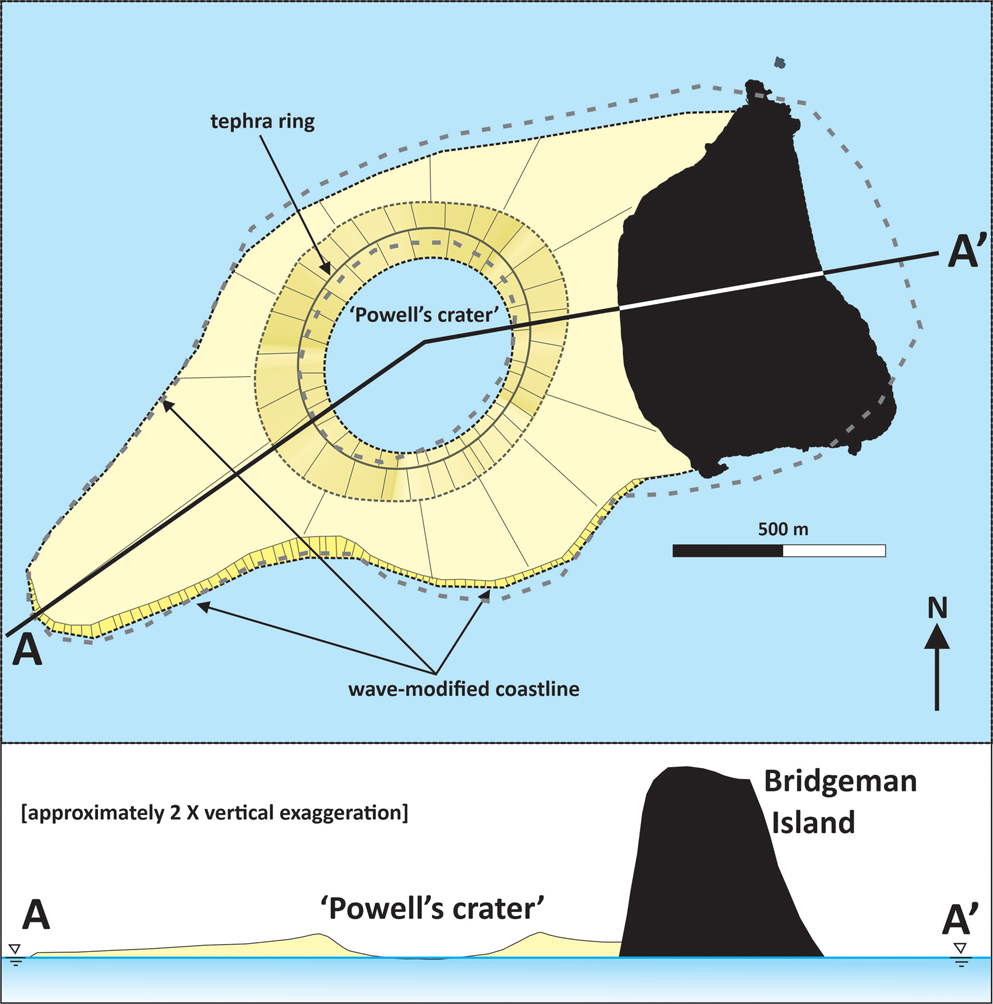
Fig. 8. Schematic reconstruction and cross-section of Bridgeman Island and the 1821 volcano. Note that without the 2× vertical exaggeration, the profile of the 1821 volcano would barely be visible above the sea. The bold grey dashed lines are the island outline and crater sketched by Powell (Reference Powell1822a) draped onto the mapped extent of modern Bridgeman Island. The coastline of the new island was already extensively modified, probably by wave action. All evidence for the 1821 volcano was removed by marine erosion, probably within a few years or at most decades of the eruption ceasing.
Tephra cones formed by hydrovolcanic explosivity in the sea are generally short-lived, typically lasting only a few months in exposed locations due to erosion by waves (Machado et al. Reference Machado, Parsons, Richards and Mulford1962, Solgevik et al. Reference Solgevik, Mattsson and Hermelin2007, Romagnoli & Jakobsson Reference Romagnoli and Jakobsson2015, Zhao et al. Reference Zhao, Mitchell, Quartau, Tempera and Bricheno2019, Plank et al. Reference Plank, Marchese, Genzano, Nolde and Martinis2020). Although islands in more protected locations can last years to many decades, they ultimately disappear below sea level and continue to be eroded down to the local wave base (usually 30–60 m; Cavallaro & Coltelli Reference Cavallaro and Coltelli2019). In water significantly deeper than 60 m, this results in the generation of a flat-topped seamount with steep flanks that extend beyond the limits of the original volcano due to the lateral transport of the unconsolidated tephra (Cavallaro & Coltelli Reference Cavallaro and Coltelli2019). This suggests that, although no physical evidence of the 1821 island exists on Bridgeman Island today, a high-fidelity swath survey conducted within 10 km west of the island might discover such a feature on the sea floor.
The location of the 1821 new island, to the west of Bridgeman Island, to which the new volcano would have been joined for a period, resembles the Surtseyan eruption of Capelinhos (Azores) in 1957–1958, in which a new submarine volcano grew into a tuff cone that became joined to the west end of the much larger island of Faial (Azores; Machado et al. Reference Machado, Parsons, Richards and Mulford1962, Cole et al. Reference Cole, Guest, Duncan and Pacheco2001). Figure 9 shows a photographic reconstruction of how Bridgeman Island and its environs might have looked in 1821 when visited by sealers such as Powell and Ames. The Capelinhos eruption also produced Strombolian products and lava effusion towards the end of its activity, but it was probably similar to the 1821 Bridgeman Island eruption in most other respects. However, unlike the 1821 eruption of Bridgeman Island, relicts of the Capelinhos volcano still exist, probably because of idiosyncrasies of the local situation (greater protection afforded by the nearby much larger island of Faial?) combined particularly with slower erosion caused by a resistant caprock of lavas. It is suggested that the 1821 eruption of Bridgeman Island was an explosive Surtseyan hydrovolcanic eruption of ‘Capelinhos type’ (i.e. grew to join up with an older island) that lasted at least 1 year. It was followed by at least 30 years of vigorous hydrothermal activity expressed as fumaroles, probably driven by a cooling shallow magma chamber that extended east under Bridgeman Island. No evidence for the 1821 volcano exists on Bridgeman Island today, but its remains may be found as a submarine seamount close to the island on its west side unless the water is so shallow that wave erosion has removed it entirely.
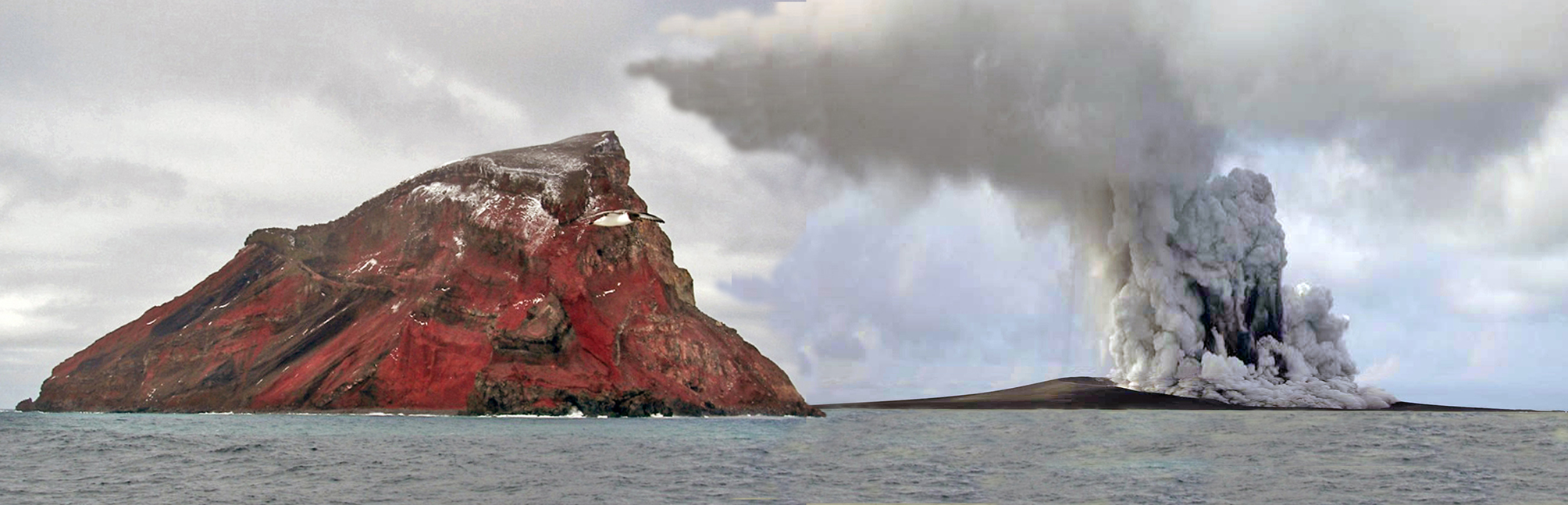
Fig. 9. Synthetic photographic view of Bridgeman Island and the new volcano, observed from the north-north-east, constructed to show how the scene might have looked when visited by sealers Ames and Powell in 1821. The eruption column on the new island at the right is of the continuous-uprush type. It is dark grey from the content of ash and its margins are collapsing to form a ring-like pyroclastic density current ('base surge') expanding out from the column base. The eruption column would probably look incandescent if seen at night. The image is a photomontage compiled from two unrelated photographs. That of Bridgeman Island is from January 2005 (image: Miguel Angel Otero Soliño, Creative Commons, Wikimedia.org), whereas the erupting island is Hunga Tonga-Hunga Ha'apai (Tonga, January 2015; image: New Zealand High Commission at Nuku'alofa, January 2022).
Conclusions
The first recorded volcanic eruption in Antarctica was attributed to Bridgeman Island, in the South Shetland Islands. It was observed by the earliest explorers (sealers) in early 1821 < 2 years after Antarctica was discovered. However, various 19th-century observations were considered vague and unreliable owing to a complete lack of physical evidence on the island. Therefore, a consensus began to develop in the 20th century that the reports probably referred to Penguin Island, a young volcano with a well-formed volcanic cone and crater situated just 60 km to the west of Bridgeman Island. However, the historical reports have recently been shown to be accurate and the locus of the volcanic event was undoubtedly Bridgeman Island. As a result of our volcanological reappraisal of the same reports, we are able to demonstrate that an explosive eruption of Bridgeman Island occurred in 1821. It lasted 1 year followed by fumarolic activity during the subsequent few decades. The vent locus was situated in the sea ~500 m west of Bridgeman Island. The activity was hydrovolcanic, characterized as Surtseyan, which formed a tuff ring edifice with a very low profile (< 30 m high, > ~2 km wide) constructed of lapilli and ash. The new volcano rapidly expanded to connect with Bridgeman Island, similar to how the well-described Surtseyan eruption of Capelinhos volcano in 1957–1958 coalesced with neighbouring Faial (Azores). However, because of its very exposed location, the unconsolidated volcanic deposits were rapidly destroyed by marine erosion, removing all visible evidence of the volcanic construct. The occurrence of active volcanism just 200 years ago followed by several decades of fumarolic activity, together with volcanism on Bridgeman Island itself dated to just a few tens of Kyr ago, implies that the prominent submarine volcanic massif underlying Bridgeman Island, known as Bridgeman Rise, should be regarded as dormant rather than extinct.
Acknowledgements
The visit to Bridgeman Island by SK was supported by the Chilean Navy and was funded by the Instituto Antártico Chileno (INACH, project number OA_05-07) and by the National Oceanic and Atmospheric Administration (NOAA) of the USA (Department of Commerce grant: NA08OAR4310867). We are also indebted to Alex Tate and Alice Fremand (British Antarctic Survey) for details of the bathymetry close to Bridgeman Island; to Bob Headland for information on sealing vessels in the South Shetland Islands in the early 1820s; and to Karoly Németh and an anonymous reviewer for their helpful comments on an early draft of the paper. Finally, the authors are also very grateful to Jeronimo López-Martínez and the New Zealand High Commission at Nuku'alofa for permission to publish their images; and to Peter Clarkson and Andy Saunders for the use of their photographs in this investigation. This study is a contribution to the aims and objectives of AntVolc (Scientific Committee on Antarctic Research (SCAR) Expert Group on Antarctic volcanism: https://scar.org/science/antvolc/home/).
Author contributions
JLS: conceptualization (lead), data acquisition (equal), data curation (equal), formal analysis (lead), funding acquisition (lead), investigation (lead), writing - original draft (lead), writing - review & editing (equal); SK: conceptualization (supporting), data acquisition (equal), data curation (equal), formal analysis (supporting), investigation (supporting), writing - original draft (supporting), writing - review & editing (equal); KW: conceptualization (supporting), data acquisition (equal), data curation (equal), formal analysis (supporting), investigation (supporting), writing - original draft (supporting), writing - review & editing (equal).