Riboflavin, also known as vitamin B2, is a water-soluble and heat-stable vitamin that plays a critical role in a wide range of biological pathways and processes, mainly serving as a coenzyme in the form of flavin mononucleotide and flavin adenine dinucleotide for a variety of flavoprotein enzymes(Reference Lykstad and Sharma1). Riboflavin is an essential nutrient for human health and needs to be included in the diet(Reference Cardoso, Libardi and Skibsted2). The rich sources of riboflavin are dairy product, meat, legumes, peas, liver, eggs and fortified grains and cereals(Reference Ross3–Reference Mahan, Escott-Stump and Raymond5). Recommended daily dietary intake of riboflavin for women and men is 1·2 mg and 1·3 mg, respectively(6). Riboflavin deficiency is prevalent all around the world, particularly in underdeveloped countries due to the low intake of dairy products and meats(Reference Mensink, Fletcher and Gurinovic7,Reference Choi, Kim and Cho8) . Relevant to clinical conditions, patients with certain types of cancers and heart disease, excessive alcohol intake, chronic diarrhoea, liver disorder and haemodialysis are at a greater risk of riboflavin deficiency(Reference Ross3,Reference Peechakara and Gupta9) . Moreover, low levels of riboflavin can cause a variety of health problems including growth retardation, sore throat, cheilosis, anaemia, renal damage, neurodegeneration, gastrointestinal tract disorders, stomatitis of the mouth and tongue and skin disorders(Reference Powers10). Riboflavin deficiency can also disrupt some biological processes such as Fe absorption, metabolism of tryptophan, mitochondrial function and metabolism of other nutrients(Reference Thakur, Tomar and Singh11).
Novel studies have addressed riboflavin-dependent cellular processes in various diseases(Reference Thakur, Tomar and Singh11). One of the processes through that riboflavin could exert a substantial effect on human health is antioxidation(Reference Ashoori and Saedisomeolia12). Free radicals are produced by all aerobic cells playing the main role in ageing as well as in chronic diseases(Reference Venkataraman, Khurana and Tai13). The toxicity of free radicals contributes to the damage of cellular macromolecules (such as DNA, proteins and lipids), inflammation, tissue damage and subsequent cellular apoptosis(Reference Ames, Shigenaga and Hagen14). Therefore, as a result, oxidative stress is related to several chronic diseases such as cancer, diabetes, CVD(Reference Romero Cabrera15–Reference Fridovich17) and some neurodegenerative disorders (such as Alzheimer’s disease or Parkinson’s disease)(Reference Chen, Guo and Kong18).The function of the antioxidant defense system of the body is to prevent any damage due to the action of free radicals(Reference Uttara, Singh and Zamboni19).
In 2014, we reviewed the existing evidence regarding antioxidant properties of riboflavin for the first time, which was published by British Journal of Nutrition(Reference Ashoori and Saedisomeolia12). Since that time, there have been several studies highlighting the antioxidant effects of riboflavin and revealing as well as novel aspects of its antioxidant function. In this review, we aim to update the current literature on riboflavin as an antioxidant nutrient. The reviewed studies are summarised in Table 1.
Table 1. Summary of the in vitro, animal and human studies included in the review

GPx, glutathione peroxidase; SOD, superoxide dismutase; TBARS, thiobarbituric acid reactive substances; GSH, reduced glutathione; MDA, malondialdehyde; GST, glutathione S-transferase; TNF-α, tumour necrosis factor alpha; 8-OHdG, 8-hydroxydeoxyguanosine; ROS, reactive oxygen species; PC, protein carbonyl; FBS, fasting blood sugar; IL2, interleukin-2; CRP, C-reactive protein; ALT, alanine transaminase; AST, aspartate transaminase.
Effect of riboflavin on antioxidant enzymes activity and GSH
Aerobic organisms need antioxidant defense systems to deal with free radicals that either are produced during aerobic respiration or may have an external origin. Antioxidant enzymes, including catalase (CAT), superoxide dismutase, glutathione peroxidase (GPx) and glutathione reductase (GR), are the main parts of antioxidant defense system that deactivate reactive radicals mainly by reducing them to a more stable compound(Reference Krishnamurthy and Wadhwani20). Glutathione peroxidase catalyses the reduction of hydrogen peroxide (H2O2)/lipid peroxide to H2O/alcohol using reduced GSH as an electron donor(Reference Krishnamurthy and Wadhwani20) (Fig. 1). Through this reaction, GSH is converted to the oxidised form (GSSG). GR reduces GSSG and recovers its antioxidant properties in the reaction that requires riboflavin in the flavin adenine dinucleotide coenzyme form(Reference Gropper and Smith4). The detailed role of riboflavin in this reaction has been explained in our previous publication(Reference Ashoori and Saedisomeolia12). Superoxide dismutase (SOD) catalyses the dismutation of superoxide ion (O2¯) to molecular oxygen (O2) and H2O2 (a less reactive species)(Reference Krishnamurthy and Wadhwani20). H2O2 could be converted to water by CAT or GPx(Reference Krishnamurthy and Wadhwani20) (Fig. 1). Considering the substantial role of antioxidant enzymes in maintaining redox homoeostasis of the cells, they are assessed as common biomarkers of the redox status of organisms(Reference Thérond, Bonnefont-Rousselot and Davit-Spraul21). As riboflavin has a key role in the glutathione redox cycle, it has been expected that riboflavin status could affect redox status, and consequently, glutathione content and activity of antioxidant enzymes. The effect of riboflavin status on antioxidant enzyme activity has been examined through several experimental studies using animal models of various diseases which have a connection with oxidative stress. Al-Harbi et al investigated the effect of riboflavin on lipopolysaccharides (LPS)-induced acute lung injury in rats(Reference Al-Harbi, Imam and Nadeem22). In this study, riboflavin administration reversed the decline in GR and GPx activities and GSH content of lung induced by LPS. In another experimental study, riboflavin therapy significantly increased the activity of SOD, CAT, GR and GSH content of kidneys in renal toxicity rat model(Reference Alhazza, Hassan and Ebaid23). The improved antioxidant enzymes activity and GSH content of tissues have been also reported after riboflavin administration in rat models of migraine (GSH, microsomal GPx)(Reference Bütün, Nazıroğlu and Demirci24), hepatotoxicity (CAT, SOD and GSH)(Reference Al-Harbi, Imam and Nadeem25,Reference Bashandy, Ebaid and Moussa26) , ulcerative colitis (SOD and GSH)(Reference Karakoyun, Ertaş and Yüksel27), anticancer drug-induced toxicity (CAT, SOD and GSH)(Reference Naseem, Hassan and Alhazza28,Reference Salman and Naseem29) , spinal cord injury (SOD and GSH)(Reference Sakarcan, Erşahin and Eminoğlu30), diabetes (GR, SOD, CAT and GSH)(Reference Alam, Iqbal and Naseem31) and abdominal aortic aneurysm (SOD)(Reference Yu, Morimoto and Yu32).

Fig. 1. The reactions catalysed by antioxidant enzymes. CAT, catalase; SOD, superoxide dismutase; GPx, glutathione peroxidase; GR, glutathione reductase; GSH, reduced glutathione; GSSG, oxidised glutathione; FAD, flavin adenine dinucleotide.
Moreover, there are several animal studies addressing riboflavin and its antioxidant properties as a factor that may affect animal growth and quality and quantity of animal products. For example, Jiang et al examined the effect of a riboflavin-deficient diet on fish flesh quality indicating that riboflavin deficiency leads to a significant decrease in antioxidant enzymes activity (GPx, GR, SOD and CAT) and GSH content in the muscle, while riboflavin sufficient diet reverses all these negative effects(Reference Jiang, Chen and Liu33). Similarly, the reduced content of GSH and decreased activity of antioxidant enzymes in the intestine (decreased GR, GPx and SOD activity)(Reference Chen, Feng and Jiang34) and gills (decreased GR, GPx, SOD and CAT activity)(Reference Chen, Feng and Jiang35) of riboflavin-deficient fish have been reported. In another study on ducks, it has been shown that a riboflavin-deficient diet decreased the activity of SOD in plasma and liver compared with those ducks fed by a riboflavin-supplemented diet(Reference Tang, Wen and Guo36).
Although in most of the reviewed studies, riboflavin has shown a positive effect on antioxidant enzymes activity, there were also some limited exceptions. Nazıroğlu et al investigated the effect of Se and Se plus riboflavin on oxidative stress in rat headache model(Reference Nazıroğlu, Çelik and Uğuz37). In this study, although GPx activity and GSH content in brain tissue showed a greater increase in Se + B2 group than the Se group, this difference was not statistically significant(Reference Nazıroğlu, Çelik and Uğuz37). Another study showed that after riboflavin administration in a rat model of headache, brain microsomal GPx activity was increased significantly; however, the increase in brain GPx was not statistically significant(Reference Bütün, Nazıroğlu and Demirci24). As the improvement in redox status of the brain has been observed in these studies, it seems that riboflavin exerts its antioxidant properties mainly through other antioxidant enzymes (which have not been assessed) or through mechanisms independent of the glutathione cycle or antioxidant enzymes, such as deactivation of reactive oxygen species (ROS) through the conversion of the reduced form of riboflavin to its oxidised form(Reference Ashoori and Saedisomeolia12). Another unexpected result was an increased activity of CAT in intestine of fish fed a riboflavin-deficient diet reported by Chen et al (Reference Chen, Feng and Jiang34). As intestine is an immune organ in fish(Reference Luo, Feng and Jiang38), the increased activity of CAT may be a protective mechanism against the impaired intestinal immune function caused by increased levels of ROS which was concurrently observed in this study.
As mentioned previously, the role of riboflavin in the glutathione redox cycle could explain how riboflavin affects oxidative stress and antioxidant enzyme activities. However, the findings of the in vitro and experimental studies indicated that the mechanism by which riboflavin influences antioxidant enzymes is not limited to its role as the cofactor for GR. These investigators have revealed new pathways through which riboflavin status may have a regulatory effect on antioxidant enzymes gene expression. In an in vitro study on human keratoconic stromal cells (keratoconus is an eye disorder that oxidative stress may be implicated in its development), an increased gene expression of antioxidant enzymes (SOD, GPx and CAT) in riboflavin-treated cells (compared with untreated cells) was reported(Reference Cheung, McGhee and Sherwin39). It has also been indicated that riboflavin deficiency decreases the mRNA level of antioxidant enzymes in muscle (GR, GPx, SOD and CAT)(Reference Jiang, Chen and Liu33), intestine (GR, GPx and SOD)(Reference Chen, Feng and Jiang34) and gills (GR, SOD)(Reference Chen, Feng and Jiang35) of fish. The findings of these studies suggest that riboflavin may exert such regulatory effect through the Nuclear Factor E2-Related Factor 2 signalling pathway(Reference Jiang, Chen and Liu33–Reference Chen, Feng and Jiang35). NRF2 is a transcriptional factor that activates the transcription of many antioxidant and detoxification enzymes and its abundance is tightly regulated at several points (i.e. transcriptional, post-transcriptional and post-translational)(Reference Tonelli, Chio and Tuveson40). It has been shown that riboflavin deficiency decreases the NRF2 mRNA level in fish(Reference Jiang, Chen and Liu33–Reference Chen, Feng and Jiang35). Moreover, the NRF2 mRNA level showed a positive correlation with the mRNA level of antioxidant enzymes in these studies(Reference Jiang, Chen and Liu33–Reference Chen, Feng and Jiang35). Riboflavin deficiency also led to a significant increase in Kelch-like ECH-associated protein 1(Keap1)(Reference Jiang, Chen and Liu33–Reference Chen, Feng and Jiang35) and decreased casein kinase 2 (CK2) mRNA level(Reference Jiang, Chen and Liu33). Keap1, as a suppressor of NRF2, binds to the NRF2 and prevents its translocation to the nucleus(Reference Ma41). On the other hand, phosphorylation of NRF2 by CK2 leads to its nuclear translocation and activation of NRF2 transcriptional function(Reference Apopa, He and Ma42). Therefore, the results of the studies on fish suggest that riboflavin deficiency decreases the antioxidant enzymes mRNA level through, at least in part, the down-regulation of NRF2 gene expression, up-regulation of Keap1 and down-regulation of CK2, resulting in the prevention of NRF2 nuclear translocation and activation (Fig. 2). Nevertheless, further research is needed to clarify the exact mechanisms through which riboflavin regulates these signalling molecules.
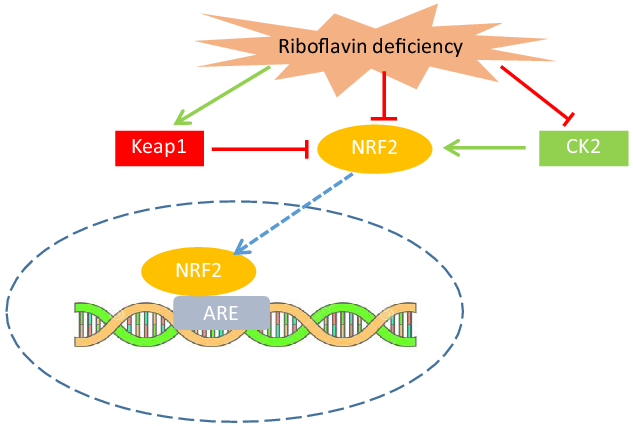
Fig. 2. Riboflavin deficiency may decrease the antioxidant enzymes activity through up-regulation of Keap1 and down-regulation of CK2, resulting in prevention of NRF2 nuclear translocation and activation. Riboflavin may also down-regulate NRF2 gene expression. CK2, casein kinase 2; NRF2, nuclear factor E2-related factor 2; Keap1, Kelch-like ECH-associated protein 1; ARE, antioxidant response element.
Finally, reviewing studies published in recent years (2014–2021) indicated that riboflavin supplementation in conditions that are accompanied by increased oxidative stress could improve the antioxidant enzymes activity; and its deficiency has shown an adverse effect on the activity of these enzymes in animals. However, to the best of our knowledge, no human research has addressed the effect of riboflavin on antioxidant enzymes activity, warranting further human studies.
Effect of riboflavin on reactive oxygen species and oxidative damage of the lipids, DNA and proteins
Reactive oxygen species (ROS) is a collective term referring to the several oxygen metabolites, which could have both exogenous and endogenous origin and include superoxide (O2 • -), hydroxyl (OH • ), peroxyl (RO2 • ), hydroperoxyl (HO2 • ) radicals and hydrogen peroxide (H2O2)(Reference Bayr43). Most of these oxygen metabolites are free radicals (H2O2 is a non-radical ROS, but can easily be converted to free radicals), which have one or more unpaired electrons making them highly reactive(Reference Halliwell and Gutteridge44). Accumulation of these compounds causes tissue damage through oxidative damage of cellular structures, that is, proteins, membrane lipids and nucleic acids(Reference Halliwell and Gutteridge44). Some specific products resulted from the oxidation of protein, lipids and DNA are assessed as the biomarkers of oxidative stress(Reference Thérond, Bonnefont-Rousselot and Davit-Spraul21).
In some experimental studies, the protective effects of riboflavin on ROS have also been reported(Reference Yu, Morimoto and Yu32,Reference Jiang, Chen and Liu33,Reference Chen, Feng and Jiang35,Reference Peraza, Guzmán and Brizuela45) . In these studies, the beneficial effect of riboflavin supplementation(Reference Yu, Morimoto and Yu32,Reference Jiang, Chen and Liu33,Reference Peraza, Guzmán and Brizuela45) and the deleterious effect of its deficiency(Reference Jiang, Chen and Liu33,Reference Chen, Feng and Jiang35) on ROS levels in different tissues have been indicated. An in vitro study also has shown that riboflavin depletion in intestinal cells could lead to overproduction of ROS in these cells(Reference Lee, Corfe and Powers46).
In our previous review, we cited studies that concluded riboflavin can alleviate lipid peroxidation(Reference Ashoori and Saedisomeolia12). Recent studies have corroborated these results(Reference Al-Harbi, Imam and Nadeem22–Reference Bashandy, Ebaid and Moussa26,Reference Naseem, Hassan and Alhazza28,Reference Salman and Naseem29,Reference Alam, Iqbal and Naseem31,Reference Jiang, Chen and Liu33–Reference Tang, Wen and Guo36) . In a recently published animal study, malondialdehyde (MDA, a marker of lipid peroxidation) is decreased after riboflavin administration in rat model of renal toxicity(Reference Alhazza, Hassan and Ebaid23). Similarly, Butun et al indicated that riboflavin significantly reduces the MDA levels in the brain of migraine rat model(Reference Bütün, Nazıroğlu and Demirci24). A significantly decreased MDA level following riboflavin therapy has also been reported in experimental models of several other diseases such as acute lung injury(Reference Al-Harbi, Imam and Nadeem22), hepatotoxicity(Reference Al-Harbi, Imam and Nadeem25,Reference Bashandy, Ebaid and Moussa26) , diabetes(Reference Alam, Iqbal and Naseem31) and cisplatin- (a chemotherapy medication) induced toxicities(Reference Naseem, Hassan and Alhazza28,Reference Salman and Naseem29) . Similarly, in other animal investigations, increased MDA has been reported in riboflavin-deficient animals which decreased following riboflavin administration(Reference Jiang, Chen and Liu33–Reference Tang, Wen and Guo36).
In addition to lipid peroxidation, riboflavin has been shown to reduce the oxidative damage of DNA(Reference Karakoyun, Ertaş and Yüksel27,Reference Yu, Morimoto and Yu32,Reference Pan, Chen and He47) and proteins(Reference Alhazza, Hassan and Ebaid23,Reference Naseem, Hassan and Alhazza28,Reference Alam, Iqbal and Naseem31) . Yu et al investigated the effect of riboflavin on abdominal aortic aneurysm formation in rats(Reference Yu, Morimoto and Yu32). This study indicated that riboflavin could significantly decrease 8-hydroxydeoxyguanosine (a biomarker for oxidative DNA damage) in aneurysm walls(Reference Yu, Morimoto and Yu32). In another experimental study reporting the protective effect of riboflavin in ulcerative colitis, the colonic 8-hydroxydeoxyguanosine level has been reported to be declined in riboflavin-treated rats compared with the control group(Reference Karakoyun, Ertaş and Yüksel27). Moreover, N-nitroso-N-methylbenzylamine (an environmental carcinogen) has caused a greater increase in oxidative DNA damage (8-hydroxydeoxyguanosine) and oesophageal tumourigenesis in riboflavin-deficient rats compared with the sufficient ones(Reference Pan, Chen and He47). Protein carbonyl, a product of protein oxidative damage, has also been shown to be decreased by riboflavin administration in the rat models of diabetes(Reference Alam, Iqbal and Naseem31), renal toxicity(Reference Alhazza, Hassan and Ebaid23) and anticancer drug-induced toxicities(Reference Naseem, Hassan and Alhazza28), while its elevated level has been reported in riboflavin-deficient fishes(Reference Jiang, Chen and Liu33,Reference Chen, Feng and Jiang34) .
Overall, results of reviewed experimental studies suggest that riboflavin could alleviate oxidative stress in the terms of lipid peroxidation and oxidative damage of DNA and proteins. However, there are a few exceptions in the findings of these studies. For example, Nazıroğlu et al. showed that supplementation with riboflavin and Se compared with Se alone caused a greater decrease in lipid peroxidation in the brain of migraine rat model, but this decrease was not statistically significant(Reference Nazıroğlu, Çelik and Uğuz37). In another study, riboflavin led to an increased lipid peroxides in different regions of rats’ brain(Reference Peraza, Guzmán and Brizuela45). Although the exact reason for such conflicting results is not clear, specific features of brain energy metabolism(Reference McKenna, Dienel, Sonnewald, Brady, Siegel and Albers48), concurrent supplementation with other antioxidants or probable technical issues may be involved in producing these results.
The observed protective effect of riboflavin against oxidative damage of lipids, proteins and nucleic acids could be mediated by the enhanced activity of antioxidant enzymes which have been reported to occur concurrent with the reduced levels of MDA(Reference Al-Harbi, Imam and Nadeem22–Reference Bütün, Nazıroğlu and Demirci24,Reference Bashandy, Ebaid and Moussa26,Reference Naseem, Hassan and Alhazza28,Reference Salman and Naseem29,Reference Jiang, Chen and Liu33,Reference Tang, Wen and Guo36) , 8-hydroxydeoxyguanosine(Reference Karakoyun, Ertaş and Yüksel27,Reference Sakarcan, Erşahin and Eminoğlu30) and protein carbonyl(Reference Alhazza, Hassan and Ebaid23,Reference Naseem, Hassan and Alhazza28,Reference Jiang, Chen and Liu33) . However, the mechanism through which riboflavin acts as an antioxidant may not be limited to its effects on antioxidant enzymes. As discussed in our previous review(Reference Ashoori and Saedisomeolia12), in some cases, riboflavin has led to a decrease in oxidative stress, while the activity of antioxidant enzymes has not increased concurrently, a finding that has been reported in recent studies as well(Reference Bütün, Nazıroğlu and Demirci24). It has been suggested that riboflavin has a direct free radical scavenging activity through conversion from reduced (dihydroriboflavin) to oxidised form(Reference Toyosaki49). NADPH-dependent methemoglobin reductase has been suggested to catalyse the reduction of oxidised riboflavin and recover its antioxidant activity(Reference Hultquist, Xu and Quandt50). Moreover, in the reviewed studies, riboflavin has been reported to increase vitamin E(Reference Bütün, Nazıroğlu and Demirci24,Reference Nazıroğlu, Çelik and Uğuz37) and vitamin C(Reference Bütün, Nazıroğlu and Demirci24) levels in the brain of rats, which highlights another path through which riboflavin may exert its antioxidant properties. The connection between GSH, vitamin C and vitamin E is illustrated in Fig. 3. GSH reduces oxidised vitamin C (dehydroascorbate) and recovers its antioxidant capability. Vitamin C, which is a powerful free radical scavenger, could regenerate active vitamin E from its radical form (tocopheroxyl radical) as well(Reference Pehlivan and Hamza51) (Fig. 3). In addition to its role in the glutathione redox cycle, riboflavin may directly act as an electron donor and reduce dehydroascorbate and tocopheroxyl radical to their active forms, the possible role which needs to be confirmed by future studies.

Fig. 3. The suggested mechanisms through which riboflavin reinforces the antioxidant effect of vitamin C and vitamin E. Riboflavin may recover the antioxidant activity of these vitamins through its role in glutathione redox cycle (A) or directly through its conversion from reduced to oxidized form (B). AA, ascorbate; DHA, dehydroascorbate; DHAR, dehydroascorbate reductase; GSH, reduced glutathione; GSSG, oxidised glutathione; GR, glutathione reductase.
Prooxidative effect of riboflavin
Although riboflavin has been widely examined as an antioxidant in experimental studies, it can also have a prooxidant effect when exposed to ultraviolet irradiation. Riboflavin itself is non-toxic in the darkness, although upon absorption of UVA and blue light, it can act as a photosensitiser(Reference Cardoso, Libardi and Skibsted2). The photoreactivity of riboflavin is due to the isoalloxazine moiety(Reference Cardoso, Libardi and Skibsted2). Through photosensitisation, the isoalloxazine ring of riboflavin absorbs energy from light and induces chemical reactions in nearby molecule through type I and type II pathways(Reference Huang and Choe52). In the type I pathway, riboflavin (in the excited triplet state) can abstract electrons or hydrogen ion from the substrates to produce radicals. However, this photosensitiser, in the type II pathway, can transfer its high energy to triplet oxygen and form singlet oxygen(Reference Huang and Choe52) and convert it to an ROS. Therefore, in the light-exposed tissues, that is, eyes and skin, riboflavin may induce oxidative damage to the macromolecules. Other dietary antioxidants such as polyphenols, vitamin E and carotenoids have been indicated to protect these tissues against riboflavin photosensitising effect by different mechanisms(Reference Huvaere, Olsen and Skibsted53,Reference Cardoso, Olsen and Skibsted54) . Polyphenols and vitamin E can quench the triplet state of riboflavin through direct electronic energy transfer(Reference Huvaere, Olsen and Skibsted53), while carotenoids scavenge-free radicals and ROS resulting from type I and type II photosensitisation(Reference Cardoso, Olsen and Skibsted54). On the other hand, photosensitising characteristics of riboflavin make it a potential target for antimicrobial/anticancer photodynamic therapy(Reference Khan, Rayis and Rizvi55,Reference Khaydukov, Mironova and Semchishen56) , a multi-stage treatment that involves light and photosensitiser to destroy abnormal cells.
Human studies
Although animal studies have focused on the riboflavin antioxidant properties and its application in the management and/or prevention of various diseases so far, human research in this area is very scarce. In a cross-sectional study examining the association between dietary B vitamins intake and urinary monomethyl-arsenic and oxidative stress marker 15-F2t-Isoprostane(Reference Howe, Li and Zens57), dietary riboflavin intake was inversely associated with urinary 15-F2t-Isoprostane in US adults; however, this association did not remain significant after adjusting for confounders. In the only available interventional research in this area, the effect of riboflavin supplementation in patients with Crohn’s disease was investigated(Reference von Martels, Bourgonje and Klaassen58). The results of this study indicated that redox status (plasma free thiols) was improved after three weeks of riboflavin supplementation in the patient with severe disease. Riboflavin also led to a significant decrease in inflammatory biomarkers and clinical symptoms of the disease(Reference von Martels, Bourgonje and Klaassen58). Although this research was a quasi-experimental study (without a control group) in which the results are just before–after comparisons, its findings highlight the possible beneficial effects of this neglected antioxidant in various conditions which have a connection with oxidative stress, the area which remains to be elucidated through further randomised controlled trials.
Clinical outcomes
The beneficial effects of riboflavin on biochemical(Reference Al-Harbi, Imam and Nadeem22,Reference Alhazza, Hassan and Ebaid23,Reference Bashandy, Ebaid and Moussa26,Reference Alam, Iqbal and Naseem31) and histopathological findings(Reference Al-Harbi, Imam and Nadeem22,Reference Alhazza, Hassan and Ebaid23,Reference Al-Harbi, Imam and Nadeem25–Reference Karakoyun, Ertaş and Yüksel27,Reference Sakarcan, Erşahin and Eminoğlu30–Reference Yu, Morimoto and Yu32) and clinical symptoms(Reference Sakarcan, Erşahin and Eminoğlu30,Reference Yu, Morimoto and Yu32) , reported concurrent with alleviated oxidative stress in animals, suggest that riboflavin antioxidant activity may lead to promising clinical outcomes in these conditions. For example, improved histopathological findings in diabetes (liver and kidney)(Reference Alam, Iqbal and Naseem31), hepatotoxicity(Reference Al-Harbi, Imam and Nadeem25,Reference Bashandy, Ebaid and Moussa26) acute lung injury(Reference Al-Harbi, Imam and Nadeem22), renal toxicity(Reference Alhazza, Hassan and Ebaid23), ulcerative colitis(Reference Karakoyun, Ertaş and Yüksel27), spinal cord injury(Reference Sakarcan, Erşahin and Eminoğlu30) and abdominal aortic aneurysm(Reference Yu, Morimoto and Yu32) have been indicated following riboflavin administration. Moreover, beneficial effects of riboflavin on renal function biomarkers in renal toxicity(Reference Alhazza, Hassan and Ebaid23), biomarkers of liver function, inflammation and lipid profile in hepatotoxicity(Reference Bashandy, Ebaid and Moussa26), biomarkers of renal and liver function and fasting blood glucose in diabetes(Reference Alam, Iqbal and Naseem31) and markers of pulmonary permeability in lung injury(Reference Al-Harbi, Imam and Nadeem22) have been reported. Furthermore, as discussed in our previous review(Reference Ashoori and Saedisomeolia12), riboflavin has also shown to have a protective effect against reperfusion oxidative injury (the tissue damage occurs after a period of ischaemia as a result of blood reperfusion) of different organs in experimental models, a finding which has been supported by the recent findings(Reference Adakul, Ertas and Çevikelli59–Reference Li, Lu and Zhao62). Therefore, the antioxidant effects of riboflavin could make it a potential therapeutic agent in mentioned conditions and other diseases in which oxidative stress is involved. However, a limited number of human studies have examined the effect of riboflavin as a therapeutic approach, warranting further human research in this regard(Reference von Martels, Bourgonje and Klaassen58).
Finally, the findings of reviewed studies provide further support for the antioxidative effects of riboflavin, although human studies are still very scarce. These effects of riboflavin could be mediated by antioxidant enzymes (through its role as an enzyme cofactor and as a gene expression regulator as well) and may be exerted through its direct free radical scavenging capacity. Riboflavin could also influence the concentration of other antioxidants such as vitamin C and vitamin E. It may also show peroxidative effect under photo illumination. Given the serious shortcoming in available human evidence, further research with stronger designs (i.e. randomised controlled trials or longitudinal studies) regarding probable beneficial effects of riboflavin in the prevention and management of diseases associated with oxidative stress are highly recommended.
Acknowledgements
This work was supported by the Tehran University of Medical Sciences, Deputy of Research.
This work did not receive any financial support.
A. S. provided the review’s concept. M. A. and N. O. drafted the manuscript. A. S. and M. A. supervised the entire process of manuscript preparation and edited the last version.
None of the authors has any conflicts of interest to declare.