Metabolisable energy (ME) in the diet is a critical factor that greatly influences the intake of all other nutrients in the poultry species(Reference Wiseman and Lewis1). Moreover, the feed efficiency, growth performance and body composition of broiler chickens are likely to be influenced by the ME intake(Reference Zhao and Kim2,Reference Ge, Wang and Ying3) . It was commonly believed that the high-ME diet for broiler chickens is produced by the addition of lipids (fats and oils) because lipids have the highest energy value compared with other nutrients(Reference Zhang, Haitao and Zhao4). However, some concerns regarding dietary lipid levels and digestibility have arisen in commercial poultry production, especially for young broiler chickens whose lipid utilisation is restricted due to the poor digestive ability and the absorption capacity(Reference Ravindran, Tancharoenrat and Zaefarian5).
Dietary fats, including poultry fat (PF) and vegetable oils, are the main energy sources in poultry feed(Reference Khonyoung, Yamauchi and Suzuki6). Increasing the fat digestibility may allow lowering supplemental lipid inclusion levels in the broiler chicken diet while maintaining the same level of performance, which ultimately results in lower feed production costs(Reference Cho, Zhao and Kim7). In general, the digestibility of lipids depends on their chemical and physical characteristics, particularly the chain length of fatty acids and their saturation degree, which influence the ME content of the diet(Reference Smink, Gerrits and Hovenier8). Ghasemi et al.(Reference Ghasemi, Shivazad and Mirzapour Rezaei9) indicated that the ratio of lower unsaturated fatty acids (UFA):SFA in the diet needs higher concentrations of bile salts (one kind of emulsifier), which are essential for micelle formation and, subsequently, lipid uptake. Hence, a higher degree of saturation or a lower UFA:SFA ratio led to poorer digestibility of the lipid source in broiler chickens(Reference Rodriguez-Sanchez, Tres and Sala10). These physiological and functional limitations of lipid digestion and absorption in different poultry species could be overcome by the supplementation of an exogenous emulsifier to the diet(Reference Liu, Ma and Krezhova11,Reference Maldonado–Valderrama, Wilde and Macierzanka12) .
Emulsifiers promote fatty acids’ incorporation into micelles and increase the digestibility of dietary lipids in the duodenum of chickens(Reference Jansen, Nuyens and Buyse13,Reference Zhang, Dudley and Davidson14) . De-oiled soyabean lecithin (DSL) is a mixture of amphiphilic phospholipids, which has good emulsification property(Reference Van Wijk, Balvers and Cansev15). Although there is scarce information on the use of DSL supplement in humans and animals, the application of some emulsifier agents in broiler chicken diets and their effects on growth performance and physiological response have been evaluated in several studies. Jansen et al.(Reference Jansen, Nuyens and Buyse13) showed that the addition of lysolecithin in the basal diet with an SFA source (pig lard) increased nitrogen retention and apparent ME of the feed during the grower period of broiler chickens. Furthermore, supplementation of a rice-bran-derived lysolecithin increased fat digestibility and improved growth performance of broiler chickens(Reference Raju, Rao and Chakrabarti16). The advantages of dietary supplementation with lysophospholipid for improving low-energy and low-nitrogenous diets were also recently observed in a study by Boontiam et al.(Reference Boontiam, Jung and Kim17). Similarly, Siyal et al.(Reference Siyal, El-Hack and Alagawany18) confirmed an increased digestibility of energy due to soyabean lecithin utilisation in the broiler chicken diets, which could be an effective strategy to decrease dietary ME levels. In the study by Alzawqari et al.(Reference Alzawqari, Nassiri Moghaddam and Kermanshahi19), an increase in jejunal and ileal villus height (VH) for a diet supplemented with an emulsifier supplementation (desiccated ox bile) was observed in broiler chickens.
To our knowledge, no previous reports are available in the literature about the effect of dietary supplementation with DSL at different dietary energy levels or different fat sources in broiler chickens.
In the present study, we used a broiler chicken model to investigate the effects of DSL supplementation as an exogenous emulsifier on growth performance, nutrient digestibility, carcass characteristics, serum biochemical parameters and jejunal morphology at different dietary ME levels provided by two fat sources (soyabean oil (SO) or PF).
Methods
All experimental procedures involving birds adhered to the guidelines of and were approved by the Animal Management and Ethics Committee (in charge of animal welfare issues) of the Arak University (Arak, Iran).
Preparation of pure de-oiled lecithin
The pure DSL, with the commercial name of BergaPur, was obtained from Berg+Schmidt GmbH & Co. KG. and is derived from the soya plant. It is manufactured in dry powder form by extracting the oil present in the natural liquid lecithin and contained 87 % of the phospholipid complex, 10 % lysophospholipid, 2 % TAG and 1 % water. The fatty acid composition of this product includes 20 % palmitic acid, 5 % stearic acid, 9 % oleic acid, 59 % linoleic acid and 7 % linolenic acid.
Experimental design and diets
A total of 800 1-d-old male Ross 308 broilers chickens (average body weight (BW): 46·4 ± 0·39 g), were purchased from a commercial hatchery and used in a 42-d feeding trial. Broiler chickens were randomly allocated to eight treatments in five pens (replicates) of twenty birds per replicate pen, so that their initial weights were similar across all treatment groups. A three-phase feeding schedule consisting of starter (0–10 d), grower (10–24 d) and finisher (24–42 d) diets was used in the present study. The experiment consisted of a 2 × 2 × 2 factorial arrangement of treatments including two concentrations of ME (starter: 12·55 MJ/kg for normal ME diet and 12·13 MJ/kg for low-ME diet; grower: ME = 12·97 MJ/kg for normal ME diet and 12·55 MJ/kg for low-ME diet; and finisher: ME = 13·39 MJ/kg for normal ME diet and 12·97 MJ/kg for low-ME diet), two fat sources (SO and PF) and two dietary levels of DSL supplementation (0 and 1 g/kg). All nutrients in experimental diets were formulated to meet or exceed the recommendations for broiler chickens, according to the Ross 308 nutrient specifications(20). The ingredients and nutrient composition of the experimental diets used during different growing periods are presented in Tables 1 and 2. The broiler chickens were provided with mash feed and tap water ad libitum during the entire experiment. All experimental groups were housed in floor pens (length 175 cm × width 170 cm) using litter top dressed with 5 cm of clean wood shavings in an environmentally controlled house. Room temperature began at 34°C for the first 3 d and was decreased gradually to 22°C until the end of the trial and the relative humidity was around 65 %. The lighting programme was standardised across all pens and consisted of 23-h light and 1-h darkness. Light source used was incandescent bulbs at light intensity of 30 lux.
Table 1. Ingredients of the experimental diets at all stages of growth

ME, metabolisable energy; SO, soyabean oil; PF, poultry fat.
* The vitamin premix supplied per kg diet: vitamin A (retinol), 3600 µg; vitamin E (α-tocopheryl acetate), 63 mg; vitamin D3 (cholecalciferol), 125 µg; vitamin K3 (menadione), 3·5 mg; thiamine, 3 mg; riboflavin, 7·5 mg; niacin, 65 mg; pantothenate, 18 mg; pyridoxine, 4·3 mg; biotin, 0·3 mg; folic acid, 2 mg; cyanocobalamin, 0·017 mg; choline chloride, 600 mg; antioxidant, 100 mg.
† The mineral premix supplied per kg diet: Mn (MnSO4·H2O, 32·5 % Mn), 120 mg; Zn (ZnO, 80·4 % Zn), 33·88 g; Fe (FeSO4·7H2O, 20·1 % Fe), 20 g; Cu (CuSO4·5H2O), 16 mg; Se (NaSeO3, 45·6 % Se), 0·3 mg, iodine (KI, 58 % iodine), 1·3 mg.
Table 2. Calculated and analysed nutrient contents of the experimental diets at all stages of growth (on as-fed basis)

ME, metabolisable energy; TSAA, total sulphur amino acids; SO, soyabean meal; PF, poultry fat; GE, gross energy.
* Mean of two samples per diet.
Growth performance measurements
BW and feed consumption for each experimental group were recorded on days 0, 10, 24 and 42. Average daily feed intake (ADFI), average daily gain (ADG) and feed conversion ratio (FCR; ADFI:ADG) were calculated. Mortalities and health status were also recorded daily to calibrate growth performance.
Sample collection and procedures
At the end of the trial (on day 42), after being fasted for 12 h, two broiler chickens from each replicate (ten birds per treatment) with BW close to the pen mean was randomly selected and weighed. Blood samples (5 ml) were collected from the wing vein in sterile test tubes without anticoagulant and incubated at 37°C for 2 h and then the serum was obtained by centrifuging at 2000 g at 4°C for 10 min and stored at –20°C. The broiler chickens were killed by cervical dislocation; then the pre-slaughter, carcass, breast, leg, liver and abdominal fat weights were recorded by trained personnel. Their relative weights were expressed as the percentage of pre-slaughter live weight.
Contents of ileum (from Meckel’s diverticulum to approximately 10 mm above the ileal–caecal junction) were collected in plastic zip bags. The ileal digesta of two birds in a replicate were pooled, after which a representative sample was immediately stored in a freezer at –20°C for subsequent determination of nutrient digestibility and apparent metabolisable energy (AMEn) corrected for nitrogen balance. A 2-cm section of mid-jejunum was also separated, flushed with distilled water to remove the contents and fixed in 10 % neutral-buffered formalin for morphological assessment.
Chemical analysis and calculations
Before chemical analysis, representative samples of feed and ileal digesta were ground in a laboratory mill to pass through a 1-mm screen. Samples were analysed for DM (method 930.15), crude protein (method 990.03) and crude fat (method 920.39), according to the standard procedures(21). Gross energy determinations of diet and ileal digesta samples were performed in an adiabatic bomb calorimeter (Parr Instrument Company) standardised with benzoic acid. The amino acid profile of the diets was analysed by an HPLC instrument (Knauer) consisting of a K-1000 controller quaternary pump and a Shimadzu fluorescence detector (RF-551). Methionine and cysteine were analysed as methionine sulfone and cysteic acid after an overnight cold performic acid oxidation before hydrolysis(Reference Moore22).
Each of the analyses for crude fat and fatty acid profile from the diet samples was conducted, according to the method previously described by Ghasemi et al.(Reference Ghasemi, Shivazad and Mirzapour Rezaei9). Individual fatty acids in the diet samples were quantified by GC (Unicam 4600, equipped with a flame ionisation detector, and a BPX70 fused silica capillary column). As an endogenous indicator, acid-insoluble ash (AIA) in both diet and ileal digesta samples were analysed to calculate the nutrient digestibility and AMEn(Reference Vogtmann, Pfirter and Prabucki23).
The digestibility of nutrients in diets was determined by the following equation:

where nutrientdiet and AIAdiet are the concentrations of nutrient and AIA in the diet (%) and Nutrientid and AIAid represent the concentrations of the same nutrient and AIA in the ileal digesta (%).
The AMEn value was calculated using the following equation(Reference Tavernari, Roza and Surek24):

where GEdiet is gross energy value in diet (MJ/kg) and GEid is the gross energy value in ileal digestibility (MJ/kg). Indigestibility factor (IF) = AIAdiet/AIAid; Ndiet is the nitrogen concentration in diet (%), Nid is nitrogen concentration in ileal digesta (%) and 34·44 is the energy equivalent (MJ/kg) of uric acid.
Blood biochemical parameters
The concentrations of glucose, TAG, total cholesterol (CHOL), HDL, LDL, total protein, albumin and uric acid in serum samples were analysed by an auto analyser apparatus (Biotecnica, BT-3000), following the instructions of the corresponding reagent kit (Pars Azmoon Co.).
Intestinal morphology
For each jejunal tissue segment, a 5-μm cross section was made using a microtome, placed on a glass slide, stained by haematoxylin–eosin and analysed with a light microscope (Olympus, CX31, Shinjuku). Three cross-sections and ten measurements per cross section were obtained. Morphological measurements of VH (from the tip of the villus to the villus–crypt junction), villus width (VW; at the middle point of the villus) and crypt depth (CD; the depth of the invagination between adjacent villi) were made using an image-analysis software (QWinPlus v. 3.1.0; Leica Cambridge Ltd.). Data from the VH and CD were used to obtain the VH:CD ratio. The villus surface area (VSA) was also calculated by the following formula: 2π × (VW/2) × VH.
Statistical analysis
The data were subjected to ANOVA for a 2 × 2 × 2 design using the General Linear Models procedures of SAS (v. 9.0; SAS Institute). The model included main effects of ME level, fat type and DSL level, and their interactions. The replicate pen of twenty broiler chickens for growth performance or two chickens for other responses served as the experimental unit for all statistical analyses. Data from mortality and carcass traits were transformed to arcsine for analysis. The results are presented as the least-square means with standard errors of the means. All statements of significance were considered as P < 0·001 or P < 0·05, with a trend between P > 0·05 and P < 0·10.
Results
Growth performance
The performance of broiler chickens fed the experimental diets is shown in Tables 3 and 4. The two-way interaction effects of ME × fat, ME × DSL, fat × DSL and the three-way interaction of ME × fat × DSL on BW and ADG were not significant (Table 3). However, the interactions between fat source and DSL supplementation were observed for FCR during the finisher (P = 0·014) and the entire experimental period (P = 0·011), indicating that the effect of DSL on FCR was more marked in broiler chickens fed on the PF-containing diets (Table 4). Broiler chickens fed high-ME diets had higher BW and ADG (P < 0·05) and lower FCR (P < 0·05) than those fed low-ME diets during the different experimental periods. At 24 d, the BW, ADG and FCR of broiler chickens receiving the SO-containing diets were better (P < 0·05) compared with birds fed on the PF-containing diets, but these improvements were not reflected during the whole experimental period (0–42 d). At 10 d, there were also tendencies that SO-containing diets resulted in higher BW (P = 0·086), ADG (P = 0·084) and lower FCR (P = 0·062) when compared with PF-containing diets. As Table 3 indicates BW of broiler chickens receiving DSL supplementation was higher at 24 d (P = 0·013) and 42 d (P = 0·072) compared with the non-supplemented birds. Dietary DSL also increased ADG in the grower (P = 0·013), finisher (P = 0·062) and overall (P = 0·071) experimental periods. Furthermore, broiler chickens supplemented with DSL had lower FCR in the grower (P = 0·005) and the overall (P = 0·019) experimental period (Table 4). ME, fat source and DSL supplementation did not affect ADFI during the whole experiment, and both two-way and three-way interactions were not significant.
Table 3. Effects of different levels of metabolisable energy (ME), various fat sources and de-oiled soyabean lecithin (DSL) supplementation on body weight (BW) and average daily gain (ADG) of broiler chickens at all stages of growth up to 42 d of age (Mean values with their standard errors; n 5)

SO, soyabean oil; PF, poultry fat.
a,b Mean values within a column with unlike superscript letters were significantly different (P < 0·05).
Table 4. Effects of different levels of metabolisable energy (ME), various fat sources and de-oiled soyabean lecithin (DSL) supplementation on average daily feed intake (ADFI) and feed conversion ratio (FCR) of broiler chickens at all stages of growth up to 42 d of age
(Mean values with their standard errors; n 5)

SO, soyabean oil; PF, poultry fat.
a,b Mean values within a column with unlike superscript letters were significantly different (P < 0·05).
Nutrient digestibility
The main effect of ME, fat source, DSL supplementation, two-way interaction effects of ME × DSL, fat × DSL and the three-way interaction of ME × fat × DSL on the ileal digestibility of DM and crude protein were not significant (Table 5). An interaction between fat sources and DSL was observed on digestibility of crude fat, which improved with DSL addition in the PF-containing diets. Supplemental DSL was also able to improve (P ≤ 0·001) the AMEn value of diet. The PF-containing diets reduced fat digestibility compared with SO-containing diets. Broiler chickens fed on low-ME diet had lower AMEn value as compared with birds fed on the normal ME diet.
Table 5. Effects of different levels of metabolisable energy (ME), various fat sources and de-oiled soyabean lecithin (DSL) supplementation on nutrient digestibility of broiler chickens at 38 d of age
(Mean values with their standard errors; n 5)

AMEn, apparent metabolisable energy; SO, soyabean oil; PF, poultry fat.
a,b Mean values within a column with unlike superscript letters were significantly different (P < 0·05).
Carcass characteristics
Neither the main effects of ME, fat source and DSL supplementation, nor their interactions on the yields of carcass, breast and legs were significant (Table 6). In contrast, the low-ME diets significantly reduced (P < 0·001) the percentage of abdominal fat and liver compared with normal ME diet. Moreover, the PF-containing diets resulted in higher abdominal fat deposition (P = 0·036) and higher liver weight (P = 0·064) when compared with SO-containing diets. There was an interaction of dietary ME and fat sources on abdominal fat percentage (P = 0·048) and a trend for an ME × fat interaction (P = 0·093) for relative liver weight.
Table 6. Effects of different levels of metabolisable energy (ME), various fat sources and de-oiled soyabean lecithin (DSL) supplementation on carcass traits* of broiler chickens at 42 d of age
(Mean values with their standard errors; n 5)

SO, soyabean oil; PF, poultry fat.
a,b Mean values within a column with unlike superscript letters were significantly different (P < 0·05).
* The values are expressed as a percentage of pre-slaughter live body weight.
Blood metabolites
Data for serum metabolites are detailed in Table 7. The main effects of dietary ME, fat source and DSL supplementation on serum glucose, TAG, total protein, albumin and globulin were not significant. Although dietary ME did not influence blood lipid profile, the broiler chickens fed on the SO-containing diets showed lower concentrations of CHOL (P = 0·018) and LDL (P = 0·003) and higher concentration of HDL (P = 0·035) compared with those fed on the PF-containing diets. Moreover, the DSL supplementation significantly decreased blood concentrations of TAG (P = 0·041), while marginally decreasing blood CHOL (P = 0·057) and LDL (P = 0·049). The two-way interaction effects of ME × fat, ME × DSL and fat × DSL and the three-way interaction of ME × fat × DSL were not significant for all blood parameters.
Table 7. Effects of different levels of metabolisable energy (ME), various fat sources and de-oiled soyabean lecithin (DSL) supplementation on blood biochemical parameters of broiler chickens at 42 d of age
(Mean values with their standard errors; n 5)
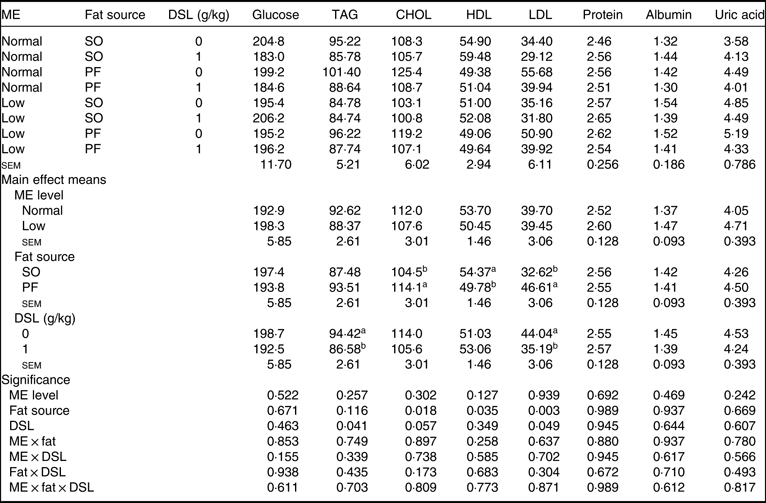
CHOL, total cholesterol; SO, soyabean oil; PF, poultry fat.
a,b Mean values within a column with unlike superscript letters were significantly different (P < 0·05).
Gut morphology
Morphological characteristics in the jejunum of broiler chickens fed the experimental diets are presented in Table 8. There was an interaction between fat sources and DSL supplementation on VH:CD (P = 0·007) in the jejunum of broiler chickens; additionally, a trend (P = 0·057) was observed for a fat × DSL interaction for the CD of the jejunum. However, the main effect of ME, two-way interaction effects of ME × fat and ME × DSL and the three-way interaction of ME × fat × DSL were not significant for all morphological parameters. Regarding the main effects of fat sources, the VH, VH:CD ratio and VSA were lower (P = 0·007, P < 0·001 and P = 0·029, respectively), whereas CD was higher (P = 0·060) in broiler chickens fed on the PF-containing diets compared with in birds fed on the SO-containing diets. Dietary DSL also increased the VH (P = 0·002) and VH:CD ratio (P < 0·001) and reduced CD (P = 0·004) in the jejunum.
Table 8. Effects of different levels of metabolisable energy (ME), various fat sources and de-oiled soyabean lecithin (DSL) supplementation on jejunum morphology of broiler chickens at 42 d of age
(Mean values with their standard errors; n 5)

VH, villus height (µm); VW, villus width (µm); CD, crypt depth (µm); VH:CD, villus height:crypt depth; VSA, villus surface area (mm2) = 2π × (VW/2) × VH; SO, soyabean oil; PF, poultry fat.
a,b Mean values within a column with unlike superscript letters were significantly different (P < 0·05).
Discussion
The present results indicate that DSL supplementation had a significant interaction with the fat type, and DSL significantly improved the FCR and fat digestibility of PF treatments, whereas no significant improvements were observed in the SO treatments. Therefore, it can be said the improvements that can be made with supplemental DSL are highly dependent on the lipid source incorporated in broiler chicken diets. Our results agree with those of Jansen et al.(Reference Jansen, Nuyens and Buyse13) who reported that supplementing exogenous emulsifier in diets enhanced the apparent digestibility of SFA to a greater extent than that of UFA in broiler chickens. Zaefarian et al.(Reference Zaefarian, Romero and Ravindran25) also reported a positive effect of lysolecithin emulsifier at the rate of 3·5 g/kg of diet on digestibility of tallow, as an SFA source. The improvement in growth performance by DSL supplementation in the PF group could be related to an increase in fat digestibility due to the emulsification property of DSL, as confirmed in the present study. Increased fat digestibility by emulsifier supplementation in broiler chickens was observed in other studies(Reference Zhao and Kim2,Reference Jansen, Nuyens and Buyse13) . Poultry fat is reported to be less easy to emulsify by the native bile salts present in the digestive tract, due to its physicochemical properties(Reference Zhang, Haitao and Zhao4,Reference Guerreiro Neto, Pezzato and Sartori26) . Dietary emulsifier could enhance the emulsification process, including the stabilisation and clearance of the lipid droplet surface by bile salts, in such a way that lipase could attach at the interphase(Reference Liu, Ma and Krezhova11,Reference Maldonado–Valderrama, Wilde and Macierzanka12) . The supplemented emulsifier may have also a beneficial role in the complex equilibrium of adsorption–desorption that is influenced by amphiphilic molecules, including bile salts, phospholipids and proteins, existing at the interphase(Reference Singh, Ye and Horne27). Therefore, it can be said that these changes induced by exogenous emulsifier could enhance the uptake of fat across the enterocyte membrane, resulting in a higher fat bioavailability of the diet.
Several studies have revealed that BW gain and feed efficiency were decreased by feeding low-energy diets, while feed intake was not affected(Reference Zhao and Kim2,Reference Ge, Wang and Ying3) . This is similar to our finding that the ADG was lower and FCR was higher in the low-ME groups than the normal ME groups, which may be due to the fact that dietary energy being more easily utilised for chicken growth. Compared with the SO treatment, a lower ADG and a higher FCR observed in the PF treatment up to 24 d might be because the amounts of lipase and bile salts produced by birds are inadequate in the early growth period(Reference Ravindran, Tancharoenrat and Zaefarian5). Ghasemi et al.(Reference Ghasemi, Shivazad and Mirzapour Rezaei9) also showed that diets with higher UFA:SFA ratio positively influenced BW gain and FCR in broiler chickens up to 28 d when dietary oils were supplemented at 50 g/kg. Hence, it can be said that the degree of fat saturation can affect the growth performance during the early and middle phases of the growth period due to better availability of energy from UFA.
In the present study, normal ME diets increased the abdominal fat deposition and liver weight at 42 d, but those values were lower when SO was used, as indicated by the interaction between ME level and fat source. Excessive fat deposition is one of the major problems for the modern poultry industry, as it not only decreases carcass yield but also causes difficulties in processing and great waste in the slaughterhouse(Reference Wang, Li and Wang28). The lower abdominal fat deposition was also in line with reduced fat contents of commercial meat cuts in broiler chickens(Reference Suksombat, Boonmee and Lounglawan29), thereby making the meat more acceptable for human consumption. Lower abdominal fat percentage found in the broiler chickens fed on the SO-containing diets compared with those receiving the PF-containing diets may be attributable to various metabolic uses of the absorbed dietary fats. It is hypothesised that the influence of dietary fat on tissue fat deposition may be associated with the fact that energy from the SFA is less readily used for metabolic purposes rather than PUFA and thus accumulates as body lipid(Reference Monfaredi, Rezaei and Sayyahzadeh30). The higher percentage of abdominal fat in the PF-fed broilers in the present study is also consistent with a higher n-6:n-3 PUFA ratio in the PF-containing diet (Table 2), possibly suggesting the elevation of the inflammatory response. It is reported that a diet high in the n-6:n-3 PUFA ratio leads to an increase in the endocannabinoid signalling system and related mediators, which result in the enhanced energy homeostasis and production of inflammatory mediators(Reference Duan, Li and Li31,Reference Simopoulos32) . There are increasing evidence that inflammatory reactions occurring in the adipose tissue contribute to greater fat storage in humans(Reference Scalia33) and broilers(Reference Khadem, Soler and Everaert34) and appear to be linked to the several metabolic syndromes(Reference Scalia33). Furthermore, the decreased HDL and increased LDL levels observed in the PF-fed broilers might be attributable to the presence of metabolically induced inflammation, leading to higher abdominal adiposity. This notion is also supported by the evidence that lowering LDL and raising HDL levels have beneficial effects on inflammation, indicating anti-inflammatory effects of HDL and/or proinflammatory properties of LDL(Reference Vaziri, Moradi and Pahl35). Since the liver is the primary site for lipogenic process, higher dietary energy content could cause excessive TAG deposition in chicken liver(Reference Sanz, Perezde Ayala and Lopez-bote36). Hence, an enhanced liver weight in normal ME groups may be associated with increased hepatic lipogenesis activity and PF could aggravate high energy-induced negative effects on hepatic lipogenesis activity and liver weight.
According to Helkin et al.(Reference Helkin, Stein and Lin37), blood TAG, CHOL, HDL and LDL are considered as key factors of lipid metabolism balance. In the present study, higher serum concentrations of CHOL and LDL and a lower concentration of HDL were found in broiler chickens fed on the PF-containing diets compared with those fed on the SO-containing diets. In general, these findings are consistent with those of recent studies(Reference Ghasemi, Shivazad and Mirzapour Rezaei9,Reference Zafari Naeini, Rezvani and Akhlaghi38) , which demonstrate modifications in blood lipid profiles of broiler chickens with the dietary fat sources differing in their degree of saturation. The decrease observed in blood TAG concentrations by replacing saturated fats in the broiler chicken diet with unsaturated fats might be related to a higher level of β-oxidation activity of UFA, resulting in a higher rate of TAG uptake from the bloodstream into body tissues(Reference Monfaredi, Rezaei and Sayyahzadeh30). This can be attractive to the consumers as a high PUFA:SFA ratio has positive health benefits for humans, particularly in protection against CVD(Reference Cao, Lu and Liang39).
In the present study, serum concentrations of TAG and CHOL were decreased by dietary DSL, which is consistent with other studies that have shown that soyabean lecithin has TAG and CHOL-lowering capacity in rats(Reference Polichetti, Diaconescu and Porte40) and humans(Reference Burgess, Neville and Rouillard41). The changes in serum lipid composition in the present study showed that DSL could improve the efficiency of the lipid utilisation in broiler chickens. Tompkins and Parkin(Reference Tompkins and Parkin42) declared that the long-term ingestion of soya phospholipids by humans could decrease serum TAG concentration via increasing hepatic lipoprotein lipase activity, resulting in an improved lipid metabolism status in the liver and consequently lower TAG levels in serum. This mechanism may be due to the faster rate of clearance of chylomicrons from the blood and slower rate of its secretion into the blood. However, further studies are required to investigate the mechanism of an emulsifier affecting serum metabolite profile.
Intestinal morphology, including VH and CD as well as the VH:CD ratio are indicators of gut(Reference Leonard, Sweeney and Bahar43). Villi are the most likely sites of nutrient absorption and increased VH and VH:CD ratio are directly related to higher digestion and absorption(Reference Leonard, Sweeney and Bahar43). Morphological analysis indicates that DSL was effective in PF-containing diets, minimising the adverse effect of a higher quantity of NEFA from PF on jejunal VH:CD ratio. This suggests that the potential effects of DSL on intestinal morphology depend on the degree of saturation of lipid incorporated into broiler chicken diets. An explanation could be that DSL supplementation acts as an emulsifier, thereby enhancing the formation of micelles and fat absorption in the intestine of broiler chickens fed on the saturated fat-containing diets(Reference Jansen, Nuyens and Buyse13). Higher fat absorption might decrease fermentation in the small intestine, leading to lower villi surface damage and consequently better growth performance(Reference Mitchaothai, Yuangklang and Vasupen44). Previous studies showed different results due to the addition of emulsifier supplementation to animal diet. For example, Boontiam et al.(Reference Boontiam, Jung and Kim17) reported that the VH, CD and VH:CD ratio in the broiler jejunum were improved as the result of supplementation with lysophospholipid (0·5, 1 and 1·5 g/kg); but with respect to the duodenal morphology, only CD was decreased by lysophospholipid. Alzawqari et al.(Reference Alzawqari, Nassiri Moghaddam and Kermanshahi19) also showed linear increases in VH, VSA and VH:CD ratio in the jejunum and ileum, but no morphological changes in the duodenum by feeding desiccated ox bile to broilers. In another study, Mitchaothai et al.(Reference Mitchaothai, Yuangklang and Vasupen44) showed no significant influence of dietary lecithin on morphological characteristics in the three different sites of the small intestine in young wild pigs. In the present study, the longer villi and VH:CD ratio of the jejunum observed in the DSL-fed broiler chickens indicates an increased nutrient absorption for optimal growth and production.
The morphological characteristics of intestinal mucosa can be modified by the metabolic activities of the intestinal microflora that have important consequences for human and animal health and well-being(Reference Reid, Sanders and Gaskins45). With regard to the influence of dietary fat source, it is reported that consuming SFA source instead of UFA source led to a higher value of pH in the intestinal contents and increased levels of pathogenic bacteria, which results in intestinal tissue destruction(Reference Józefiak, Świątkiewicz and Kierończyk46).
In conclusion, the low-ME diets negatively affected growth performance but had no significant effects on blood metabolites and gut morphology. In contrast, the dietary fat types, SO or PF, had no effect on the overall performance of broiler chickens but influenced abdominal fat deposition, fat digestibility, serum lipid concentrations and jejunum morphology. Dietary DSL supplementation could promote feed efficiency and gut health when applied to broiler chicken diets containing saturated fat source such as PF, probably due to increasing its utilisation efficiency and consequently increasing its ME. However, the inclusion of 0·1 % DSL to the low-energy diet could not exert an extra beneficial effect in terms of growth performance and physiological traits.
Acknowledgements
The authors greatly acknowledge the Schmidt+Berg representative in Iran for supplying DSL.
This research was financially supported by Arak University (grant no. 97–43).
The authors’ contributions are as follows: L. M. designed the experiment and conducted most of the experiments; H. A. was in charge of research idea and writing the manuscript. I. H. participated in project implementation and manuscript revision; M. H. M. performed the data analysis. All authors have read and confirmed the final version of the manuscript.
The authors declare that they have no financial or personal conflicts of interests related to this research.