Arginine, a conditionally essential amino acid, exerts an important role in physiological function. Besides serving as an essential building block for tissue protein, arginine is a substrate for several non-protein, N-containing compounds, including agmatine, proline and glutamate. Thus, arginine plays vital roles in the regeneration of adenosine triphosphate, cell proliferation, vasodilatation, neurotransmission and Ca release(Reference Wu and Morris1–Reference Wu, Bazer and Davis3). Additionally, arginine metabolism generates several functional substances, such as creatine, polyamines and NO(Reference Nieves and Langkamp-Henken4, Reference Li, Yin and Li5). Polyamines are essential for proliferation and differentiation of cells, including lymphocytes(Reference Minarini, Milelli and Tumiatti6). Creatine is an essential energy source for muscle contraction and neurons. Furthermore, NO plays an important role in physiological events (e.g. ovulation and placental growth) and in pathological conditions (virus and bacterial infection)(Reference Battegay7).
The versatile beneficial roles of arginine are not limited to physiological function; arginine also plays a vital role in the immune system(Reference Minarini, Milelli and Tumiatti6). Results of recent studies indicate that arginine stimulates the functional activities of different cell types in the immune system, including natural killer cells, macrophages, lymphokine-activated killer cells, T and B cells(Reference Li, Yin and Li5, Reference Bronte, Serafini and Mazzoni8, Reference Peranzoni, Marigo and Dolcetti9). For example, animal experiments have shown that arginine could increase thymic weight, as well as the number and reactivity of thymic lymphocytes(Reference Barbul, Wasserkrug and Sisto10, Reference Tan, Li and Kong11). Likewise, Reynolds et al. (Reference Reynolds, Daly and Shou12) also reported that arginine supplementation enhanced thymic weight, splenocyte mitogenesis, natural killer cell activity and macrophage cytotoxicity. In addition, arginine is also a critical factor affecting the differentiation of pro-B to pre-B cells in bone marrow and the release of these cells from the bone marrow(Reference Park, Hayes and Garlick13, Reference de Jonge, Kwikkers and te Velde14). These immunostimulatory effects of arginine are more pronounced in immunocompromised hosts after trauma, surgical stress or immunosuppression by HIV or other viruses(Reference Ralph, Kelly and Anstey15, Reference Ren, Yin and Liu16). For example, Kirk et al. (Reference Kirk, Regan and Wasserkrug17) reported that arginine therapy resulted in an increased number and mitogenic reactivity of extrathymic T cells in athymic mice. Ren et al. (Reference Ren, Yin and Liu16) also found that dietary arginine supplementation partially decreased the reproductive failure caused by porcine circovirus type 2 infection in the mouse model.
Collectively, arginine has versatile physiological and immunological functions. However, there is no information in the scientific literature regarding an immunomodulatory effect of arginine in vaccine-immunised mammals. Thus, the present study was conducted to evaluate the immunostimulatory effects of dietary l-arginine supplementation in mice immunised with an inactivated Pasteurella multocida vaccine. The adjuvant effects of arginine were also compared with those of an oil adjuvant.
Materials and methods
Preparation of the bacterium and inactivated vaccine
The P. multocida serotype A (CQ2) strain used in the present study was isolated from clinically infected cattle. The main reason for the use of this strain is that we previously obtained a large amount of relevant data, including the optimal vaccine dose and LD50 (median lethal dose), the methods to detect serum antibodies, which greatly facilitated the conduct of the present study. The bacteria isolated were cultured in Martin's broth agar medium containing 5 % equine serum. The pathogenicity of the isolate was tested in healthy mice by intraperitoneal inoculation of a 2 d culture, with all mice being succumbed to infection between 36 and 48 h post-administration. The bacteria re-isolated from these infected mice were used to prepare the inactivated vaccine. The bacterial inoculum was identified to be P. multocida serotype A using the PCR method and biochemical characteristics. Before the animal experiments, the inactivated whole-cell vaccine of P. multocida serotype A was prepared. Briefly, bacterial colonies isolated from the liver of mice were cultured in Martin's agar at 37°C overnight, and then an isolated colony from the overnight static culture was transferred to 100 ml of Martin's broth for incubation at 37°C for 12 h at 85 rpm. Then, P. multocida serotype A strains were inactivated by the addition of 0·4 % formalin to the culture mix (109 colony-forming units (cfu)/ml), followed by incubation at 37°C for 24 h with continuous agitation.
Experimental design
A total of ninety-five female Kunming (KM) mice (body weight 18–22 g) were obtained from the Laboratory Animal Center of Central South University. Mice were housed in a pathogen-free mouse colony (temperature, 20–30°C; relative humidity, 45–60 %; lighting cycle, 12 h/d) and had free access to food (a standard rodent diet) and drinking-water. The animals were randomly divided into six groups (n 19 per group): (1) mice were fed a diet supplemented with 0·2 % arginine (0·2 % arginine+basal diet; Ajinomoto, Inc.) from day 0 and immunised with the inactivated vaccine at a dose of 109cfu at days 15 and 20 (V-0·2 %R group); (2) mice were fed a diet supplemented with 0·5 % arginine from day 0 and immunised with the inactivated vaccine at a dose of 109cfu at days 15 and 20 (V-0·5 %R group); (3) mice were fed a diet supplemented with 1·0 % arginine from day 0 and immunised with the inactivated vaccine at a dose of 109cfu at days 15 and 20 (V-1·0 %R group); (4) mice were immunised with the inactivated vaccine and received an oil adjuvant at a dose of 109cfu at days 15 and 20 (V-adjuvant group); (5) mice immunised with the same volume of PBS at days 15 and 20 (the control group). At day 34, all of the mice were challenged by an intraperitoneal injection of P. multocida serotype A (CQ2) at a dose of 4·4 × 105cfu (2LD50). From each group, ten mice were used to calculate the survival rate, and the other animals were killed to collect serum at 36 h post-infection for the determination of cytokine levels and antibody titres. At day 34, serum antibody titres in all groups of mice were also measured before challenge. The present study was performed according to the guidelines of the Laboratory Animal Ethical Commission of the Chinese Academy of Sciences.
Analysis of serum cytokines
Serum cytokines, including IL-1β, IL-6, IL-8 and TNF-α, were measured using ELISA kits in accordance with the manufacturer's instructions (Cusabio Biotech Company Limited). An aliquot (100 μl) of the sample or standard was added to duplicate wells of a microtitre plate, which had been pre-coated with an appropriate antibody. The buffer was used as a negative control. The plate was incubated for 2 h at 37°C. A 100 μl sample of biotin antibody was added to each well after the removal of the liquid from each well and incubated for 1 h at 37°C. The wells were washed three times with 200 μl of a washing buffer. A 100 μl quantity of horseradish peroxidase–avidin was then added to each well for 1 h at 37°C. After the final wash, an aliquot (90 μl) of the 3,3′,5,5′-tetramethylbenzidine substrate was added and incubated for 30 min in the dark at 37°C. The reaction was stopped with 50 μl of the terminating solution and absorbance of the solution measured at 450 nm.
Serum glutathione peroxidase
Serum glutathione peroxidase (GSH-PX) was measured using spectrophotometric kits in accordance with the manufacturer's instructions (Nanjing Jiancheng Biotechnology Institute).
Antibody detection by ELISA
ELISA were used for the detection of antibodies. Before detection, the antigens were prepared. A 10 ml bacterial culture was crushed by an ultrasonic wave, and then diluted with a bicarbonate buffer (pH 7·4). A ninety-six-well plate was coated by antigens (100 μl of the prepared antigens) and incubated for adsorption at 4°C for 16 h. The serum collected from the mice was used as the first antibodies, and the peroxidase-conjugated goat anti-mouse IgG (Jackson ImmunoResearch) as the secondary antibody. Absorbance was read at 450 nm.
Statistical analysis
All statistical analyses were performed using SPSS 17.0 software (SPSS, Inc.). Data are expressed as means with their standard errors. Multiple comparisons were performed using the Student–Newman–Keuls method. Survival rates of mice were evaluated using Kaplan–Meier analysis and Proc Lifetest of SAS 9.2 (SAS Institute Inc.). Differences were considered significant at P< 0·05.
Results
Cytokine profile and glutathione peroxidase
Concentrations of IL-1β, IL-6, IL-8 and TNF-α in serum were measured at 36 h after infection. As indicated in Table 1, immunisation with the inactivated vaccine, regardless of adjuvant or arginine supplementation, increased (P< 0·05) the circulating level of IL-1β, in comparison with the control group, while no difference was found between immunisation with the adjuvant and arginine supplementation groups, or among all the arginine supplementation groups. Unlike IL-1β levels, concentrations of IL-6, IL-8 and TNF-α in the serum of the control group were higher than those in the other groups, but no difference was detected among the arginine groups (Table 1). Furthermore, immunisation along with dietary supplementation with 0·5 % arginine (P< 0·01) decreased serum GSH-PX levels, when compared with the control group. However, GSH-PX activity in the serum of the V-1·0 %R and V-adjuvant groups was lower than those in the V-0·2 %R and control groups, but no difference was detected between the V-0·2 %R and control groups (Fig. 1).
Table 1 Concentrations of IL-6, IL-8 and TNF-α in the serum of control and arginine-supplemented mice after challenge with Pasteurella multocida serotype A (CQ2) at a dose of 4·4×105colony-forming units (Mean values with their standard errors, n 6)

V-0·2 %R, mice immunised with an inactivated vaccine and fed diets supplemented with 0·2 % arginine; V-0·5 %R, mice immunised with an inactivated vaccine and fed diets supplemented with 0·5 % arginine; V-1·0 %R, mice immunised with an inactivated vaccine and fed diets supplemented with 1·0 % arginine; V-adjuvant, mice immunised with an inactivated vaccine and an oil adjuvant; control, mice immunised with PBS.
a,bMean values within a column with unlike superscript letters were significantly different (P< 0·001).
* Mean value was significantly different from that of control (P< 0·05).
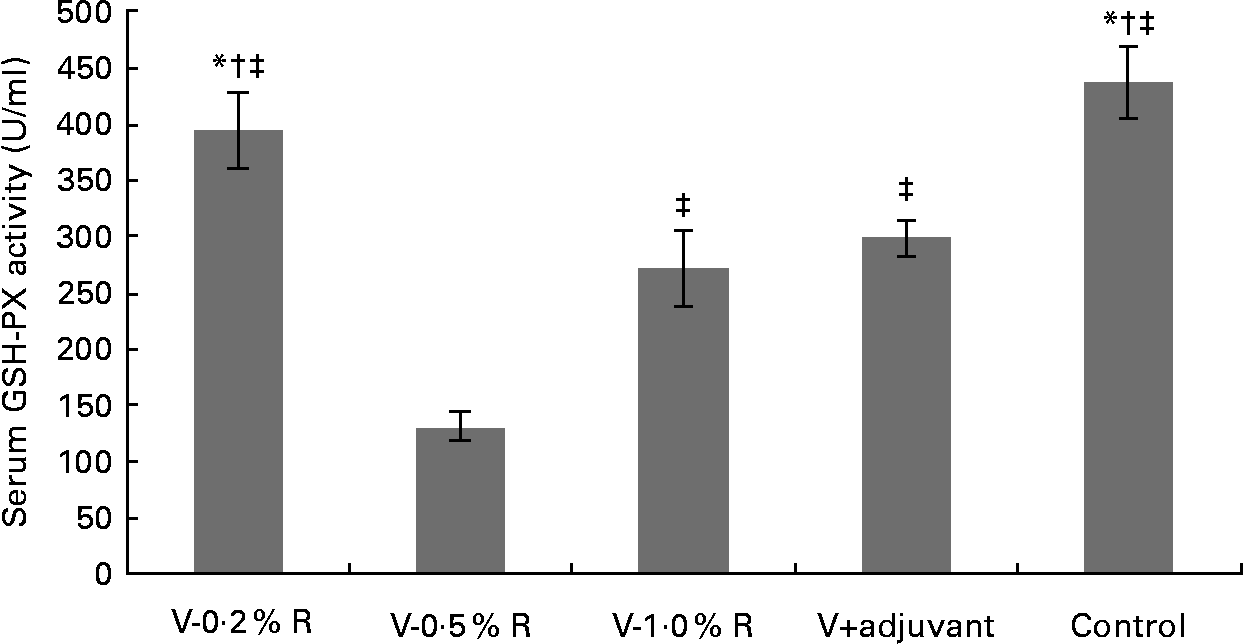
Fig. 1 Serum glutathione peroxidase (GSH-PX) levels in control and arginine-supplemented mice after challenge with Pasteurella multocida serotype A (CQ2) at a dose of 4·4 × 105 colony-forming units. Mice were immunised with an inactivated vaccine and fed diets supplemented with 0·2 % arginine (V-0·2 %R group), 0·5 % arginine (V-0·5 %R group), 1·0 % arginine (V-1·0 %R group), an oil adjuvant (V-adjuvant group), or mice immunised with PBS as the control group. Values are means, with standard errors represented by vertical bars (n 6). * Mean values were significantly different from those of the V+adjuvant group (P< 0·01). † Mean values were significantly different from those of the V-1·0 %R group (P< 0·01). ‡ Mean values were significantly different from those of the V-0·5 %R group (P< 0·01).
Serum antibody titres
Serum antibody titres against P. multocida serotype A were measured before and after being challenged with P. multocida serotype A. As shown in Table 2, serum antibody titres in the V-R and V-adjuvant groups were much higher (P< 0·001) than those in the control group. Meanwhile, immunisation with an inactivated vaccine, along with dietary 0·2 % arginine supplementation, increased (P< 0·05) serum antibody titres, when compared with immunisation with the inactivated vaccine and an oil adjuvant.
Table 2 Serum antibody titres against Pasteurella multocida serotype A in different groups of control and arginine-supplemented mice (Mean values with their standard errors, n 6)
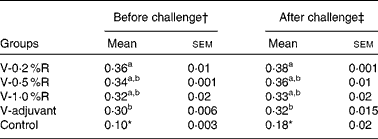
V-0·2 %R, mice immunised with an inactivated vaccine and fed diets supplemented with 0·2 % arginine; V-0·5 %R, mice immunised with an inactivated vaccine and fed diets supplemented with 0·5 % arginine; V-1·0 %R, mice immunised with an inactivated vaccine and fed diets supplemented with 1·0 % arginine; V-adjuvant, mice immunised with an inactivated vaccine and an oil adjuvant; control, mice immunised with PBS.
a,bMean values within a column with unlike superscript letters were significantly different (P< 0·05).
* Mean value was significantly different from those of the other groups in the column (P< 0·01).
† Before infection with P. multocida serotype A.
‡ At 36 h post-infection with P. multocida serotype A.
Survival rates
The survival rates were calculated every day after infection with P. multocida serotype A. All mice were dead in the control group between 36 and 48 h post-administration, and two mice were dead in both the V-1·0 %R and V-adjuvant groups. Interestingly, no death was observed in the V-0·2 %R and V-0·5 %R groups. However, Proc Lifetest of SAS 9.2 analysis showed that no difference was observed among the arginine-supplemented and adjuvant groups, while the survival rates in the vaccine group were significantly higher than those in the control group (Fig. 2).
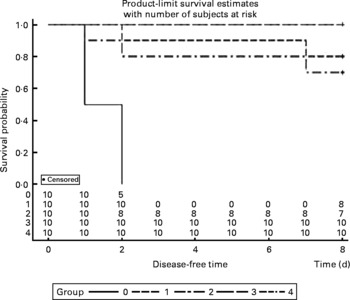
Fig. 2 Rates of mortality in control and arginine-supplemented mice after challenge with Pasteurella multocida serotype A (CQ2) at a dose of 4·4 × 105colony-forming units. Mice were immunised with an inactivated vaccine and fed diets supplemented with 0·2 % arginine (V-0·2 %R group), 0·5 % arginine (V-0·5 %R group), 1·0 % arginine (V-1·0 %R group), an oil adjuvant (V-adjuvant group), or mice immunised with PBS as the control group (n 10 per group). 0, Control; 1, V-adjuvant; 2, V-1·0 %R; 3, V-0·5 %R; 4, V-0·2 %R.
Discussion
The present study was conducted to evaluate the immunomodulatory effects of dietary l-arginine supplementation in mice immunised with an inactivated P. multocida vaccine. Serum antibody titres were measured to assess the stimulatory effects of arginine on the systemic humoral immune response. A substantial increase was observed in all the mice immunised with the inactivated P. multocida vaccine, in comparison with mice immunised with PBS. Meanwhile, the most promising results were that the antibody titres in the V-0·2R group were much higher than those in the V-adjuvant group at days 34 and 36 h post-challenge. These compelling results indicate that dietary supplementation with 0·2 % arginine increased serum antibody titres when mice were immunised with the inactivated P. multocida vaccine. Similar positive results also were observed in the chicken by Tayade's group, who reported that antibody titres in the intermediate plus+l-arginine group (chickens vaccinated with a live intermediate plus strain of the infectious bursal disease virus vaccine and supplemented with 2 % l-arginine) were higher than intermediate plus-vaccinated chickens(Reference Tayade, Jaiswal and Mishra18, Reference Tayade, Koti and Mishra19). Additionally, Tan et al. (Reference Tan, Li and Kong11) found that concentrations of total IgG and IgM in serum were higher in piglets supplemented with 0·4–0·8 % arginine, compared with unsupplemented piglets. Arginine was involved in the differentiation of pro-B to pre-B cells in the bone marrow and in the release of these cells from the bone marrow(Reference Park, Hayes and Garlick13, Reference de Jonge, Kwikkers and te Velde14). Besides these reports, the catabolism of arginine by NO synthase is enhanced to produce NO after stimulation by helper T cells type 1 (Th1) cytokines, and NO is crucial for the host defence against viruses, bacteria, fungi, malignant cells, intracellular protozoa and parasites(Reference Peranzoni, Marigo and Dolcetti9, Reference Nathan and Hibbs20–Reference Raines, Kang and Hibbs22). Moreover, arginine regulates the secretion of insulin, growth hormone, prolactin and insulin-like growth factor-I, which could mediate an NO-independent effect of arginine on immune function(Reference Li, Yin and Li5, Reference Newsholme, Brennan and Rubi23). For example, prolactin enhances the expression of antigen-presenting MHC class II molecules(Reference Li, Yin and Li5). These mechanisms may explain why higher antibody titres were observed in the V-0·2 %R group, compared with the control group. Although there were reports on the effects of arginine on vaccine-immunised chickens, no study has been conducted in this regard with a mammalian species. To our knowledge, the present study is the first to indicate that dietary arginine supplementation can enhance specific antibody titres in the serum of mice. This compelling result supports the view that arginine supplementation confers a useful adjuvant effect in vaccine-immunised mammals.
Cytokines actively participate in the immune response(Reference Praveena, Periasamy and Kumar24). IL-1β is one of the pivotal early-response pro-inflammatory cytokines that, through up- or down-regulation of other cytokines, enables organisms to respond to infectious non-self challenges and induce a cascade of effects leading to inflammation(Reference Dinarello25). IL-6 plays a very complex role in biological events, including immune responses, haematopoiesis, and modulation of endocrine and nervous systems(Reference Biffl, Moore and Moore26, Reference Naugler and Karin27). IL-8 is often associated with inflammation by acting preferentially on neutrophils(Reference Baggiolini, Loetscher and Moser28), whereas TNF-α plays a key role in immune regulation, increasing lymphoid development, cell development, cell proliferation, differentiation, activation and death(Reference Che'en, Xu and Liu29, Reference Smyth and Johnstone30). The results of the present study indicate that concentrations of IL-6, IL-8 and TNF-α in the serum of the control group were much higher than those in the other groups. These observations are consistent with the previous finding that pro-inflammatory cytokines were increased in mice after challenge with P. multocida serotype A1, while high antibody titres in other groups could protect mice from high levels of pro-inflammatory cytokines(Reference Praveena, Periasamy and Kumar24). However, serum IL-β levels in the V-R and V-adjuvant groups were much higher than those in the control group. The underlying mechanisms are unknown, but we observed that dietary arginine supplementation increased serum IL-1β levels in mice infected with porcine circovirus (W Ren, Y Yin and G Wu, unpublished results). Notably, serum GSH-PX levels in the control group were higher than those in the V-adjuvant group. This result suggests that immunisation resulted in an unfavourable effect on cellular oxidative defences. Similarly, our previous research also indicated that serum malondialdehyde levels were markedly increased, while serum total superoxide dismutase levels were decreased after immunising mice with DNA vaccines (W Ren, Y Yin and G Wu, unpublished results). However, the exact relationship between the immune system and the antioxidant system is not fully understood and warrants further investigation. Notably, we found that dietary supplementation with 0·2 % arginine beneficially reversed this unfavourable decrease in serum GSH-PX levels when mice were immunised with the inactivated P. multocida vaccine. These results are consistent with the previous notion that arginine regulates cellular redox status and plays a role in oxidative defences either directly or through its metabolic products(Reference Wu and Morris1, Reference Flynn, Meininger and Haynes31–Reference Wu, Fang and Yang33). Chander & Chopra(Reference Chander and Chopra34) also reported that arginine administration restored depleted renal antioxidant enzymes in ischaemia–reperfusion-induced rats.
The mortality in each group of mice was calculated after the immunised mice were infected with P. multocida serotype A (CQ2) at a dose of 4·4 × 105cfu. No death was observed in both the V-0·2 %R and V-0·5 %R groups, while two mice died in the V-adjuvant groups. Also, two dead mice were observed in the V-1·0 %R group, which indicated that an overdose of arginine supplementation maybe has an adverse effect on the host, as reported for cardiovascular and gastrointestinal systems(Reference Chander and Chopra34). At least, large amounts of NO produced by inducible NO synthase could exert a deleterious effect on mammalian cells and mediate the pathogenesis of many diseases, including the autoimmune destruction of pancreatic B cells in type 1 diabetes mellitus, arthritis, glomerulonephritis, inflammatory bowel disease and neurological disorders(Reference Flynn, Meininger and Haynes31). Furthermore, several studies have documented that an overdose of NO was responsible for the inhibition of T-cell proliferation and function by blocking the phosphorylation and activation of key signalling molecules, including Janus kinase 1 and 3, signal transducers and activators of transcription 5, extracellular regulated protein kinases and protein kinase B at the level of IL-2 receptor signalling(Reference Bronte, Serafini and Mazzoni8, Reference Bingisser, Tilbrook and Holt35, Reference Mazzoni, Bronte and Visintin36).
Collectively, the results of the present study demonstrate that dietary arginine supplementation confers significant immunostimulatory effects in mice immunised with an inactivated P. multocida vaccine. Thus, arginine can be used as an adjuvant for administration of vaccines in animal production. Additionally, based on serum antibody titres and mortality, optimal supplemental doses of arginine are 0·2–0·5 % in the mouse model.
Acknowledgements
This study was jointly supported by the MATS-Beef Cattle Yak system (CARS-38), the NSFC (31110103909), the National Basic Research Project (2012CB124704), the State Key Laboratory of Food Science and Technology, the Nanchang University project (SKLF-TS-201108), the Ministry of Science and Technology of the People's Republic of China (2010GB2D200322) and the Hunan Provincial Natural Science Foundation of China (10JJ2028, 2011NK2011). The authors have no conflicts of interest to declare. Y. Y. was in charge of the whole trial. W. R. and L. Z. conducted the animal experiment and wrote the manuscript. N. L., Y. W., Y. P., J. D., L. C., G. L. and G. W. assisted with the animal trial and chemical analyses.