Introduction
Sorghum is an important food and feed crop in Australia and grows well in marginal environmental conditions (Chapman et al. Reference Chapman, Cooper and Hammer2002). In 2021, sorghum was grown on 0.51 million ha in Australia with an average grain yield of approximately 2,600 kg ha−1 (ABARES 2022). Sorghum is typically planted in the late spring to the early summer (October to December) in eastern Australia, but water stress and high temperatures during grain filling (December) and plant maturation (January) stages reduce its yield potential (Muchow et al. Reference Muchow, Hammer and Vanderlip1994).
Recently, it was found that winter-optimized sorghum genotypes planted in the late winter (end of August) have the potential to avoid heat stress at the flowering stage (Singh et al. Reference Singh, Nguyen, McLean, Chapman, Zheng, Oosterom-Van and Hammer2017). Grain producers in eastern Australia have shown interest in winter-planted sorghum cultivation, as it helps to avoid terminal heat and water stress (Eyre et al. Reference Eyre, Serafin and Rodriguez2019). However, weed management in winter-planted sorghum is a concern because of the lack of registered herbicide options.
Worldwide, weed competition has been found to reduce yields by 40% to 80% in summer-grown sorghum crops (Burnside and Wicks Reference Burnside and Wicks1969; Lagoke et al. Reference Lagoke, Shebayan, Adeosun, Iwafor, Olukosi, Adu, Olufajo, Emechebe, Zaria, Adeoti, Onyibe and Chobe1990; Bararpour et al. Reference Bararpour, Hale, Kaur, Singh, Tseng, Wilkerson and Willett2019). Winter-planted sorghum will also face competition from winter weeds, such as sterile oats. In Australia, sterile oats are a major weed in winter crops, with reported yield losses of up to 80% (Mahajan and Chauhan Reference Mahajan and Chauhan2021a). A recent study in eastern Australia revealed that cohorts of sterile oats germinated from March to October (Mahajan and Chauhan Reference Mahajan and Chauhan2021b). Therefore winter-planted sorghum could face competition from sterile oats, and information on the control of sterile oats in winter-planted sorghum is limited.
Recently, imidazolinone (IMI)-resistant sorghum was introduced in Australia (Pacific Seeds, Toowoomba, Australia). With the frequent planting of IMI-resistant sorghum and repeated use of IMI herbicides, weeds may experience high selection pressure for resistance to these herbicides (Boutsalis et al. Reference Boutsalis, Gill and Preston2016; Dilipkumar et al. Reference Dilipkumar, Burgos, Chuah and Ismail2018). Preemergence (PRE) herbicides, such as atrazine, pyroxasulfone, terbuthylazine, and triallate, are widely used for the control of weeds in sorghum crops (Bagavathiannan et al. Reference Bagavathiannan, Everman, Govindasamy, Dille, Jugulam and Norsworthy2018); however, the sensitivity of IMI-resistant sorghum to these herbicides is unknown, particularly when planted in the winter growing season.
Limited data are available on the potential use of the aforementioned herbicides for the control of sterile oats in winter-planted IMI-resistant sorghum. Therefore the objectives of this research were to (1) identify alternate herbicide options for sterile oats control in winter-sown IMI-resistant sorghum and (2) assess the crop injury levels due to herbicides.
Materials and Methods
Herbicide evaluation experiments were conducted in field trials from late winter (August) to midsummer (January) in 2020 to 2021 and 2021 to 2022 at the Gatton Research Farm (27.5514°S, 152.3428°E) of the University of Queensland, Australia, to evaluate sterile oats control in sorghum. The 15 treatments (Table 1) were evaluated and arranged in a randomized complete block design with three replicates.
Table 1. Herbicides used in the study and their manufacturers.
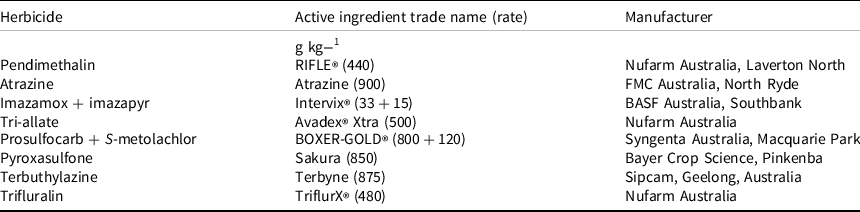
The IMI-resistant Elite Sentinel IG sorghum variety (Pacific Seeds) was planted on August 30 and August 27 in 2020 and 2021, respectively, without using any seed safener. The soil type at the experimental site was medium clay with 1.3% organic matter and a pH of 6.8. Before sorghum planting, a fine seedbed was prepared in the field using a rotary cultivator. After seedbed preparation, a sterile oats seedbank (60 seeds m−2) was established by mixing the seeds with sand and then hand broadcasted uniformly over each plot. These seeds were then mixed in the top 5 cm of the soil using a shallow tillage operation. The dimensions of each plot were 6.5 m in length by 1.4 m in width. Just before crop sowing, PRE herbicides were applied through a CO2-pressurized backpack sprayer using a delivery rate of 100 L ha−1 (200 kPa and 3.0 km h−1). The sprayer had four flat-fan nozzles (AirMix® 110015, model 25611, TeeJet® Technologies, BA Group, North Geelong, Australia) that were fixed with a 50-cm spacing. PRE herbicides were sprayed before crop sowing and incorporated by irrigation.
After the application of PRE herbicides, sorghum was planted at a density of 10 plants m−2 at a 35-cm row spacing using a cone planter. The planter had a low-soil disturbance disk system; therefore the incorporation of PRE herbicides by the sowing operation was limited. The field was surface irrigated using a sprinkler system to apply 50 mm of irrigation immediately after sowing and kept as rainfed afterward. The postemergence (POST) herbicide imazamox + imazapyr was applied 14 d after crop emergence using the same herbicide application techniques described for PRE treatment.
Crop emergence data were recorded from two 1-m crop row lengths within each plot 14 d after planting. A percentage scale from 0% to 100% was used for the visual assessment of crop phytotoxicity on a plot basis at 42 d after planting (0 = no injury; 100% = complete crop death).
Sterile oats density, seeds per square meter, and biomass were determined at crop maturity in two quadrats (50 × 50 cm) placed along the two central rows of each plot. In each quadrat, sterile oats plants were counted, collected by cutting at the base, then placed in oven bags and oven-dried at 70 C for 72 h. For weed seed count, empty and nonempty funicles (stalks of seed on which the seed sits) of each sterile oats panicle that occurred in the quadrat area of 50 × 50 cm were counted. The sorghum crop was harvested in the third week of January (2021 and 2022) in each season using a combine harvester. Harvested grains from each plot were weighed, and the moisture content in sorghum grains was accessed using an HE 50 (PFEUFFER, Kitzingen, Germany) moisture meter. The grain yield of sorghum was adjusted to 12% moisture using the following formula:
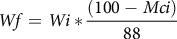
where Wf is the final weight of grains adjusted at 12% moisture, Wi is the initial weight of grains, and MCi is the moisture content in the grains recorded with a moisture meter.
The analysis of variance (ANOVA) indicated a nonsignificant interaction between years and herbicide treatments (Supplementary Table 1); therefore the data were pooled over 2 yr using the software CPCS1 developed by Punjab Agricultural University, Ludhiana, India, and verified with GenStat, 21st edition (VSN International, Hemel Hempstead, UK). The years were considered to be random effects and weed control treatments were considered to be fixed effects in mixed model analysis. Before ANOVA, data were also validated for meeting the assumptions of normality and equal variance. Treatment means were separated with the use of Fisher’s protected least significant difference (LSD) test. Unless indicated otherwise, after ANOVA, means were separated using LSD at α = 0.05.
Results and Discussion
Crop phytotoxicity was observed in plots treated with pendimethalin (27%), prosulfocarb + S-metolachlor (8% to 11%), and trifluralin (58% to 59%) applied alone or in sequential treatments (Table 2). Sorghum crop phytotoxicity was higher (P < 0.05) in PRE treatments for trifluralin and pendimethalin compared with the nontreated control, atrazine, and imazamox + imazapyr treated plots (Table 2). There was no evidence of crop phytotoxicity from other herbicide treatments (Table 2).
Table 2. Effect of herbicide treatments on crop phytotoxicity score (45 d after planting) and crop emergence (14 d after planting) in field experiments conducted at Gatton, Australia (mean of 2 yr). a,b
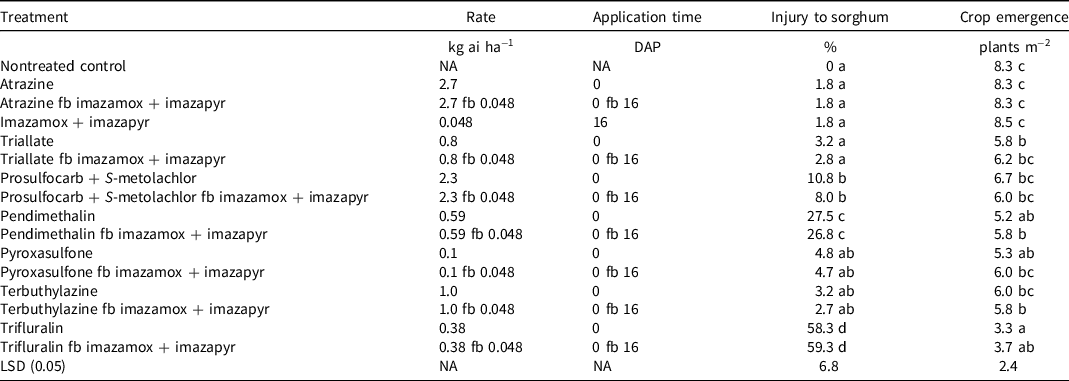
a Abbreviations: DAP, days after planting; fb, followed by; LSD, least significant difference; NA, not applicable.
b Means within the same column followed by the same letter are not significantly different (P = 0.05).
All herbicide-treated plots had lower (P < 0.05) sterile oats densities and biomass when compared to the nontreated control, except for pendimethalin, trifluralin, and prosulfocarb + S-metolachlor PRE only (Table 3). Sterile oats were completely controlled when plots were treated with atrazine followed by (fb) imazamox + imazapyr and triallate fb imazamox + imazapyr. Atrazine and imazamox + imazapyr reduced sterile oats density by 95% compared with nontreated control. Triallate and terbuthylazine reduced sterile oats density by 61% and 65%, respectively, compared with nontreated control (Table 3).
Table 3. Effect of herbicide treatments on sterile oats density, biomass, and seed number and grain yield of sorghum in field experiments conducted at Gatton, Australia (mean of 2 yr). a,b
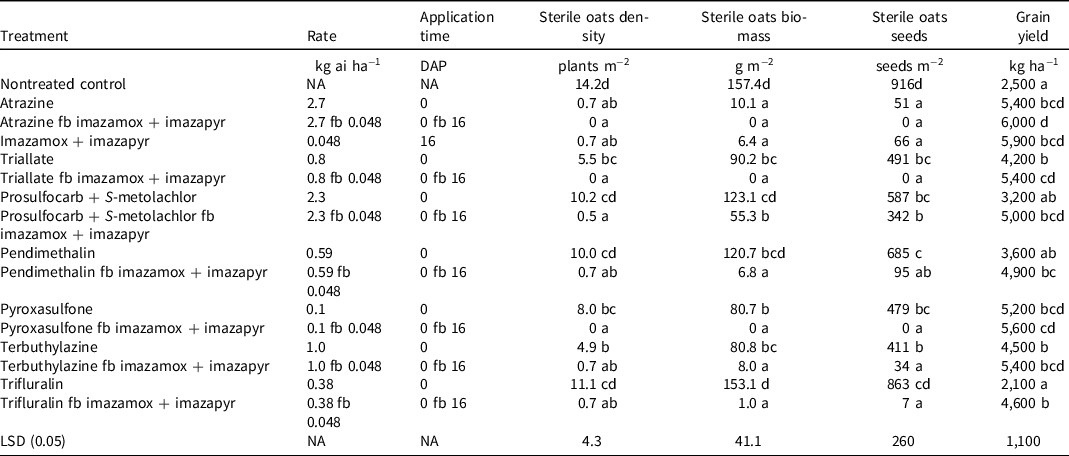
a Abbreviations: DAP, days after planting; fb, followed by; LSD, least significant difference; NA, not applicable.
b Means within the same column followed by the same letter are not significantly different (P = 0.05).
Atrazine is a well-known broad-spectrum herbicide used for weed control in summer sorghum (Geier et al. Reference Geier, Stahlman, Regehr and Olson2009; Bagavathiannan et al. Reference Bagavathiannan, Everman, Govindasamy, Dille, Jugulam and Norsworthy2018). The current study suggests that atrazine can also be used to control sterile oats in winter-planted sorghum. In Australia, 116 populations of Avena spp. (mixture of wild and sterile oats) were tested in 2005 for their susceptibility to imazapic and imazapyr (IMI), and all tested populations were found to be susceptible (Owen and Powles Reference Owen and Powles2009). Later on, these authors evaluated 101 populations of Avena spp. (mixture of wild and sterile oats) in 2010 against imazamox + imazapyr and found 20% survival in one population, whereas other populations were susceptible to this herbicide (Owen and Powles Reference Owen and Powles2016). These observations suggest that atrazine and IMI herbicides could be very effective in controlling sterile oats in winter sorghum fields. However, repeated use of atrazine in IMI-resistant sorghum may also evolve atrazine-resistant weeds due to its high selection pressure. Currently, 74 weed species have been documented as resistant to triazine herbicides (Heap Reference Heap2022). Therefore it is advisable to use atrazine and the IMI group of herbicides in rotation for sustainable weed control.
In the nontreated control plot, sterile oats biomass was 157 g m−2, which was similar (P > 0.05) to the biomass in plots treated with pendimethalin, trifluralin, and prosulfocarb + S-metolachlor applied alone (Table 3). Application of atrazine and imazamox + imazapyr reduced the sterile oats biomass by >95% compared with nontreated control. However, triallate, pyroxasulfone, and prosulfocarb + S-metolachlor applied alone reduced the biomass of sterile oats by 43%, 22%, and 49%, respectively, compared with nontreated control.
For treatments in which the PRE mixture of imazamox + imazapyr, except prosulfocarb + S-metolachlor (atrazine fb imazamox + imazapyr, triallate fb imazamox + imazapyr, pendimethalin fb imazamox + imazapyr, terbuthylazine fb imazamox + imazapyr, trifluralin fb imazamox + imazapyr), was applied in the sequential spray, sterile oats biomass was reduced by >95% compared with nontreated control (Table 3).
In nontreated control plots, sterile oats produced 916 seeds m−2, which was similar (P > 0.05) to the seed production in plots treated with pendimethalin (685 seeds m−2) and trifluralin (863 seeds m−2) (Table 3). Application of atrazine and imazamox + imazapyr reduced sterile oats seed production by 95% and 93%, respectively, compared with nontreated control. The poor control of sterile oats in sorghum fields may allow sterile oat plants to produce seeds. High seed production and shattering ability of sterile oats plants may increase the weed seed bank in the sorghum field and increase its infestation in subsequent years.
Sorghum grain yield was 6,000 kg ha−1 in the atrazine PRE and imazamox + imazapyr POST treatment; however, the yield in this treatment was similar (P > 0.05) to those in plots treated with a single application of atrazine PRE (5,400 kg ha−1; 116% higher yield than nontreated control), imazamox + imazapyr POST (5,900 kg ha−1; 136% higher yield than nontreated control), and pyroxasulfone PRE (5,200 kg ha−1; 108% higher yield than nontreated control) (Table 3). There were similarly (P > 0.05) low grain yields in the nontreated control (2,500 kg ha−1), prosulfocarb + S-metolachlor (3,200 kg ha−1), pendimethalin (3,500 kg ha−1), and trifluralin (2,100 kg ha−1) PRE treatments.
Sorghum yield was 68% higher (P < 0.05) in the triallate-alone treatment compared to nontreated control (Table 3). Yield increased by 116% compared to nontreated control when imazamox + imazapyr POST followed the triallate PRE application. Similarly, sorghum yield also increased by 125% compared to nontreated control when pyroxysulfone PRE was followed by imazamox + imazapyr POST. Yield did not improve in the plots treated with the PRE application of pendimethalin, trifluralin, and prosulfocarb + S-metolachlor compared with nontreated control. However, when these PRE herbicide applications were followed by imazamox + imazapyr, yields increased by 96%, 84%, and 100%, respectively, compared with nontreated control. Although crop emergence was poor with pendimethalin and trifluralin treatments (Table 2), improved weed control with POST imazamox + imazapyr in these treatments reduced crop–weed competition to result in higher yields than when this PRE treatment was used alone. It needs to be noted here that the reduced sorghum emergence in pendimethalin, trifluralin, prosulfocarb, and S-metolachlor treatments will restrict yield potential regardless of any subsequent weed control with imazamox + imazapyr.
This study further suggests that sterile oats can emerge in winter-planted sorghum, impose competition on the crop by limiting growth resources (water and nutrients), and reduce grain yield significantly. The best herbicide treatment (atrazine fb imazamox + imazapyr) had a substantially higher yield (140%) than nontreated control.
The sterile oats density of 14 plants m−2 (nontreated plot) produced 916 seeds m−2 that could lead to further reinfestation of this weed (Table 2; Figure 1), as most seeds shattered at the crop harvesting stage (visual observation). Results revealed that sterile oats can be effectively controlled with the application of atrazine (PRE), pyroxasulfone (PRE), and imazamox + imazapyr (POST) and may help in reducing weed seedbank by prohibiting fresh seed production.
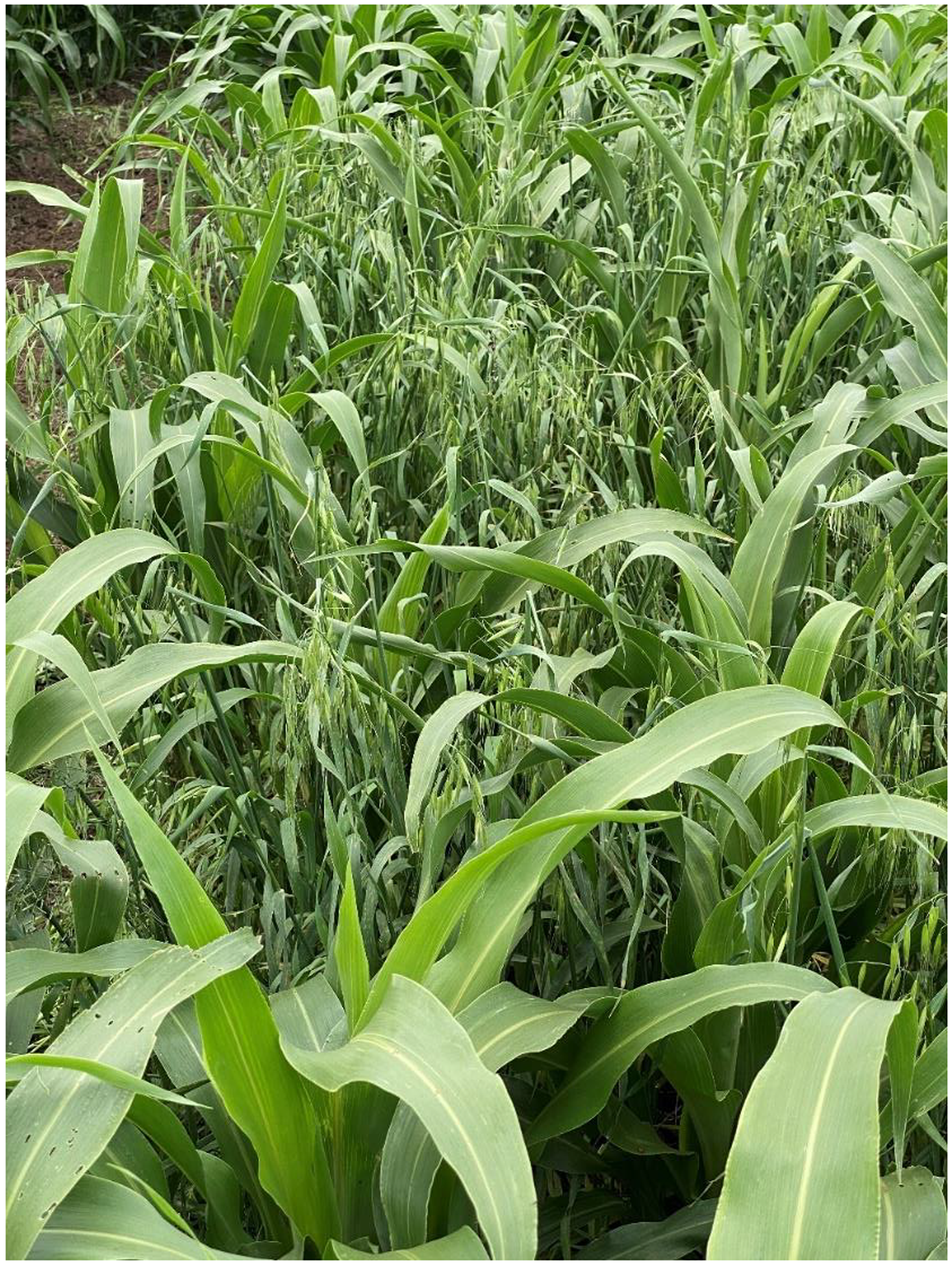
Figure 1. Sterile oats infestation in a nontreated plot of winter-planted sorghum at Gatton Research Farm of the University of Queensland, Australia, 2021 to 2022.
In conclusion, application of atrazine at 2.7 kg ai ha−1 PRE and imazamox + imazapyr at 0.048 kg ai ha−1 POST provided effective control of sterile oats in winter-planted sorghum and resulted in the highest sorghum yield. However, there are concerns, such as an increase in the risk of shifting weed flora and resistance buildup when making the application of pre-mix herbicides that contain two acetolactate synthesis–inhibiting active ingredients (Boutsalis et al. Reference Boutsalis, Kleemann, Gill and Preston2014). Further research is needed to evaluate the residual herbicides’ carry-over effects on subsequent rotational crops. This study suggests that atrazine could also be used as an alternative herbicide for weed control in IMI-resistant sorghum. Alternative use of atrazine in IMI-resistant sorghum could reduce the selection pressure of IMI herbicides on sterile oats. Herbicide-resistant weeds are a worldwide problem, and repeated use of a single herbicide may increase the evolution of herbicide-resistant weeds. One of the best approaches for reducing the selection pressure on weeds is to rotate herbicides with different modes of action (Riar et al. Reference Riar, Norsworthy, Steckel, Stephenson, Eubank, Bond and Scott2013).
Supplementary material
To view supplementary material for this article, please visit https://doi.org/10.1017/wet.2022.90
Acknowledgments
This research received no specific grant from any funding agency or the commercial or not-for-profit sectors. The authors declare no conflicts of interest.