Leishmania parasites
Leishmaniasis is a neglected disease in tropical and subtropical regions caused by the intracellular parasites from the genus Leishmania and transmitted by bites of infected sand fly vectors (Torres-Guerrero et al., Reference Torres-Guerrero, Quintanilla-Cedillo, Ruiz-Esmenjaud and Arenas2017). Cutaneous leishmaniasis (CL), mucocutaneous leishmaniasis (MCL) and visceral leishmaniasis (VL) are three important forms of this disease (Torres-Guerrero et al., Reference Torres-Guerrero, Quintanilla-Cedillo, Ruiz-Esmenjaud and Arenas2017). Metacyclic promastigotes, the infective forms of these parasites after the bite, are phagocytosed by the host macrophages, turning later into amastigotes to proliferate inside these cells and cause progressive infection (Frank et al., Reference Frank, Marcu, Petersen, Weber, Stigloher, Mottram, Scholz and Schurigt2015; Rashidi et al., Reference Rashidi, Kalantar and Hatam2018). The host immune cells activate macrophage killing programme to eliminate the intruder, however the ability of Leishmania parasite to evade or suppress the host immune response positively correlates with infection progression (Gupta et al., Reference Gupta, Oghumu and Satoskar2013). Although the chemotherapy is considered the most effective way to treat leishmaniasis, due to the presence of antimonial drug resistance and side effects of such compounds, there is an increased need of novel therapeutic targets and new fully effective drugs available for treatment (Pérez-Victoria et al., Reference Pérez-Victoria, Bavchvarov, Torrecillas, Martínez-García, López-Martín, Campillo, Castanys and Gamarro2011; Rashidi et al., Reference Rashidi, Nguewa, Mojtahedi, Shahriari, Kalantar and Hatam2020b, Reference Rashidi, Fernández-Rubio, Manzano-Román, Mansouri, Shafiei, Ali-Hassanzadeh, Barazesh, Karimazar, Hatam and Nguewa2021). Identifying the parasite strategies to alter the macrophage defence mechanisms and to survive within these cells, could bring new insights and suggest novel therapeutic targets for leishmaniasis (Rabhi et al., Reference Rabhi, Rabhi, Ben-Othman, Rasche, Daskalaki, Trentin, Piquemal, Regnault, Descoteaux and Guizani-Tabbane2012; Rashidi et al., Reference Rashidi, Kalantar, Nguewa and Hatam2020a; Kalantar et al., Reference Kalantar, Manzano-Román, Ghani, Mansouri, Hatam, Nguewa and Rashidi2021). Accordingly, since microRNAs (miRNAs, miRs) are involved in most of the mechanisms relevant to the parasite pathogenicity and survival in the infected host cells, their inhibition could be a new therapeutic approach to control parasite proliferation and immune evasion (Hashemi et al., Reference Hashemi, Sharifi, Masjedi, Tolouei, Hashemi, Mortazavidehkordi, Mohaghegh, Hashemi and Hejazi2018a).
miRNAs
miRNAs are small non-coding RNAs, approximately containing 22–24 nucleotides, synthesized by enzymes called RNA polymerase II and III. In the nucleus, through out a maturation process the primary miRNAs are converted into miRNA precursor and then translocated to the cytoplasm where they mediate gene inhibition through miRNA-RISC complex (Fig. 1).
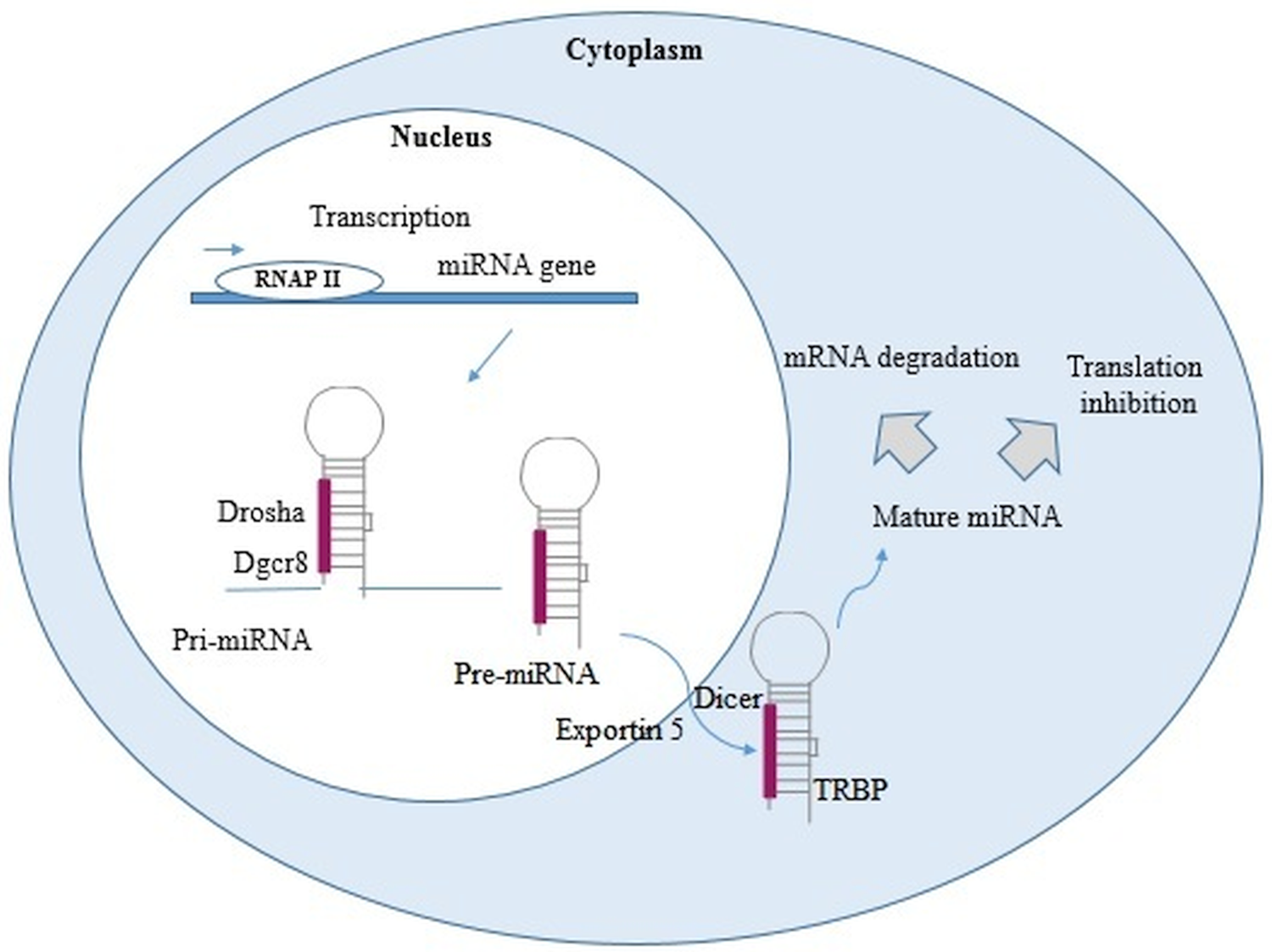
Fig. 1. miRNA biogenesis. In the nucleus, RNA polymerase II or III transcribed miRNA genes into primary miRNAs (pri-miRNAs). Pri-miRNAs, after being processed by Drosha and DiGeorge syndrome Critical Region 8 (DGCR8), become into miRNAs precursor (pre-miRNAs). These pre-miRNAs are exported into the cytoplasm by exportin 5 and Ras-related nuclear protein (Ran) Guanosine-5′-triphosphate (RanGTP), then cleaved by Dicer, and finally turned into two single-stranded RNA (ssRNAs). The ssRNAs interact with RNA-induced silencing complex (RISC), protein complex [formed by Argonaute 2, Dicer, and transactivation response RNA binding protein (TRBP)]. The gene inhibition, mediated by miRNA-RISC complex, may take place through a site-specific cleavage, or by enhancing mRNA degradation or through translational inhibition (Cai et al., Reference Cai, Kolluru and Ahmed2017; Treiber et al., Reference Treiber, Treiber and Meister2019; Condrat et al., Reference Condrat, Thompson, Barbu, Bugnar, Boboc, Cretoiu, Suciu, Cretoiu and Voinea2020; Matsuyama and Suzuki, Reference Matsuyama and Suzuki2020).
miRNAs regulate gene-expression post-transcriptionally by modulating mRNA degradation and altering protein levels. These processes are considered the primary molecular mechanism responsible for some pathological processes including cancer (Thomson et al., Reference Thomson, Newman, Parker, Morin-Kensicki, Wright and Hammond2006). Remarkably, both innate and adaptive immune responses are affected by miRNAs, leading to their effects on the clinical symptoms of different diseases (Raisch et al., Reference Raisch, Darfeuille-Michaud and Nguyen2013; Cheng et al., Reference Cheng, Sharples, Scicluna and Hill2014). For instance, miRNAs exert important functions in many aspects of the regulation of immune cell function by targeting inflammation-associated genes, including toll-like receptors (TLRs). Parasite recognition by TLRs leads to macrophage activation and control of Leishmania infection via the orchestrated production of pro-inflammatory and microbicidal effector molecules (Gallego et al., Reference Gallego, Golenbock, Gomez and Saravia2011). As a pathogenicity strategy, Leishmania parasites are able to change the TLR signalling pathways by modulating the expression level of miRNAs in infected-macrophages to subvert the host immune responses (Muxel et al., Reference Muxel, Acuña, Aoki, Zampieri and Floeter-Winter2018a). Furthermore, miRNAs can also act as physiological ligands of specific TLRs and initiate the signalling cascade of immune responses (He et al., Reference He, Jing and Cheng2014; Bayraktar et al., Reference Bayraktar, Bertilaccio and Calin2019).
miRNAs and diseases
The ability of miRNAs to usurp different signalling pathways and consequently change the cellular response and the outcome of diseases is a hotspot in medical research science nowadays (Fig. 2) (Yang and Wang, Reference Yang and Wang2016; Butterworth, Reference Butterworth2018; Barbu et al., Reference Barbu, Condrat, Thompson, Bugnar, Cretoiu, Toader, Suciu and Voinea2020; Gorabi et al., Reference Gorabi, Kiaie, Sathyapalan, Al-Rasadi, Jamialahmadi and Sahebkar2020; Lei et al., Reference Lei, Chen, Zhang, Shan, Ye, Liang, Sun, Liao, Zhu and Chen2020; Ghafouri-Fard et al., Reference Ghafouri-Fard, Abak, Shoorei, Mohaqiq, Majidpoor, Sayad and Taheri2021). Furthermore, miRNAs have been also suggested as valuable biomarkers in the treatment, diagnosis, and prognosis (Ali Syeda et al., Reference Ali Syeda, Langden, Munkhzul, Lee and Song2020; Chakraborty et al., Reference Chakraborty, Sharma and Sharma2020; Chandan et al., Reference Chandan, Gupta and Sarwat2020; Condrat et al., Reference Condrat, Thompson, Barbu, Bugnar, Boboc, Cretoiu, Suciu, Cretoiu and Voinea2020; Matsuyama and Suzuki, Reference Matsuyama and Suzuki2020; Tribolet et al., Reference Tribolet, Kerr, Cowled, Bean, Stewart, Dearnley and Farr2020).
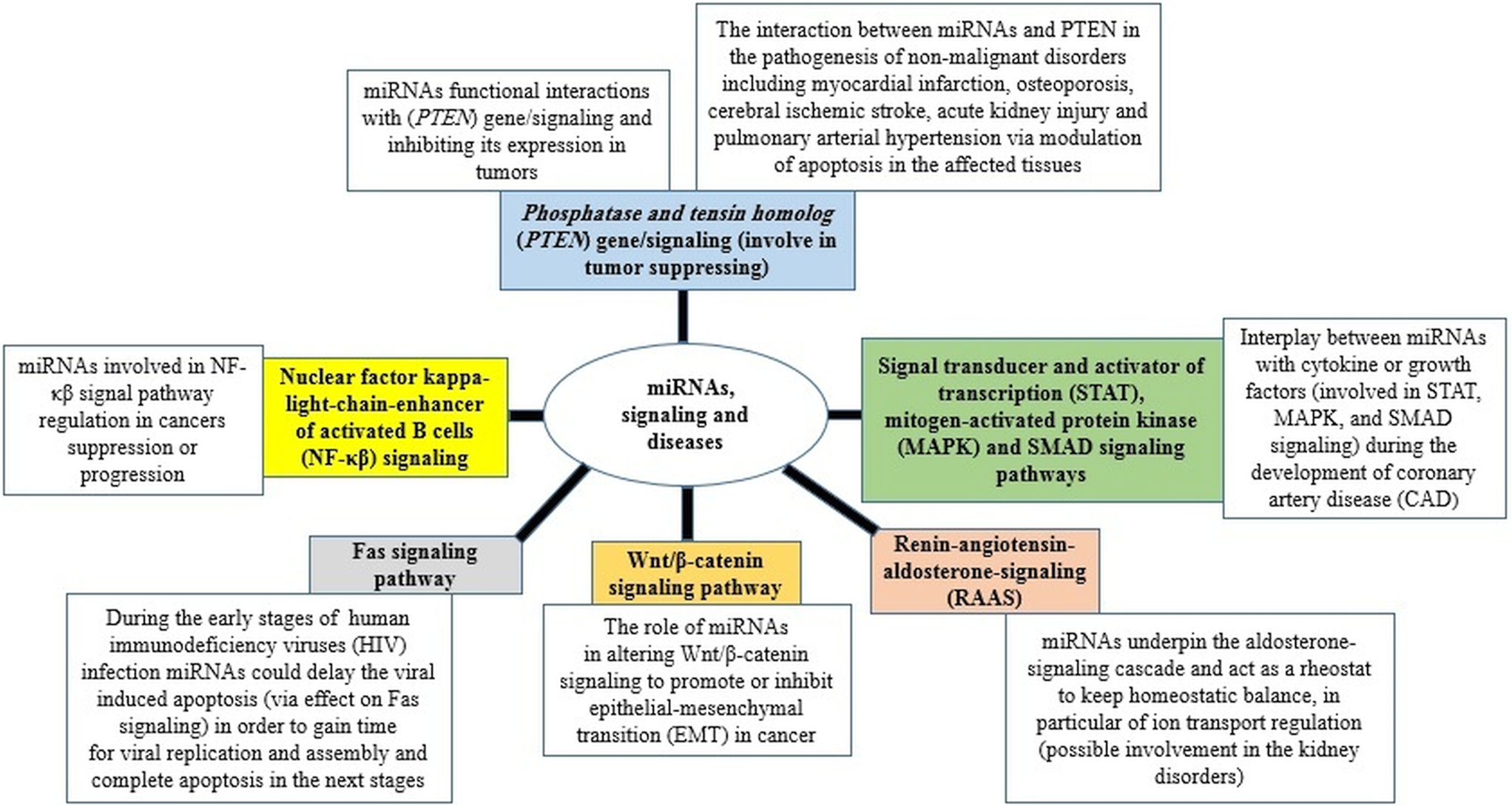
Fig. 2. miRNAs in signalling pathways and diseases.
In this sense, many diseases have been associated with changes in the expression level of miRNAs, including systemic rheumatic diseases, nervous system disorders, sepsis, cardiovascular disease and different type of cancers such as breast, ovarian, cervical forms (Ceribelli et al., Reference Ceribelli, Yao, Dominguez-Gutierrez, Nahid, Satoh and Chan2011; Abd-Aziz et al., Reference Abd-Aziz, Kamaruzman and Poh2020; Ali Syeda et al., Reference Ali Syeda, Langden, Munkhzul, Lee and Song2020; Condrat et al., Reference Condrat, Thompson, Barbu, Bugnar, Boboc, Cretoiu, Suciu, Cretoiu and Voinea2020). In addition, several investigations have recently demonstrated changes in circulating miRNAs in response to different infectious diseases, increasing the possibility for a new diagnostic tool (Acuña et al., Reference Acuña, Floeter-Winter and Muxel2020; Tribolet et al., Reference Tribolet, Kerr, Cowled, Bean, Stewart, Dearnley and Farr2020), even before the pathogen could be directly distinguished and prior to the onset of seroconversion (Stewart et al., Reference Stewart, Marsh, Jenkins, Gantier, Tizard, Middleton, Lowenthal, Haining, Izzard and Gough2013; Biswas et al., Reference Biswas, Haleyurgirisetty, Lee, Hewlett and Devadas2019). Thus, alterations in blood miRNA profiles have been associated with pathogens or pathologies such as Hendra virus (Stewart et al., Reference Stewart, Marsh, Jenkins, Gantier, Tizard, Middleton, Lowenthal, Haining, Izzard and Gough2013), tuberculosis (Zhang et al., Reference Zhang, Guo, Fan, Li, Wei, Yang, Jiang, Chen, Wang and Liu2013) and Ebola (Duy et al., Reference Duy, Koehler, Honko, Schoepp, Wauquier, Gonzalez, Pitt, Mucker, Johnson and O'Hearn2016), human immunodeficiency virus (HIV) (Biswas et al., Reference Biswas, Haleyurgirisetty, Lee, Hewlett and Devadas2019) and malaria (Li et al., Reference Li, Huang, Li, Li, Wang, Wang, Li, Zheng and Zou2018), including differentiating complicated and uncomplicated Plasmodium vivax malaria (Kaur et al., Reference Kaur, Sehgal, Kumar, Sehgal, Bansal and Sultan2018). Interestingly, miRNAs have also been highlighted in influenza infections (Scheller et al., Reference Scheller, Herold, Kellner, Bertrams, Jung, Janga, Greulich, Schulte, Vogelmeier and Lohmeyer2019) and rhinoviruses (Hasegawa et al., Reference Hasegawa, Pérez-Losada, Hoptay, Epstein, Mansbach, Teach, Piedra, Camargo and Freishtat2018). Therefore, the potential diagnostics use of miRNAs with other respiratory viruses, such as the severe acute respiratory syndrome coronavirus 2 (SARS-CoV-2), is completely reasonable. Two recently works have reviewed the literature on the potential role of cellular miRNAs in the SARS-CoV-2-host interplay as a therapeutic option in coronavirus disease 2019 (COVID-19) patients (Fani et al., Reference Fani, Zandi, Ebrahimi, Soltani and Abbasi2021; Zhang et al., Reference Zhang, Amahong, Sun, Lian, Liu, Sun, Lou, Zhu and Qiu2021). The main conclusions of these two works are that miRNAs can inhibit the SARS-CoV-2 infection by interfering in various biological processes; blocking the angiotensin-converting enzyme 2 (ACE2) or the transmembrane protease serine 2 (TMPRSS2) as well as regulate the cytokine storm. Also, miRNAs-based therapeutics could be used in the nanovaccines.
miRNAs during host−parasite interactions
Host−pathogen interactions lead to modifications in signalling and physiological processes in host cells that induce the miRNA-mediated post-transcriptional regulation of genes involved in different cellular mechanisms such as the inflammatory response during the induction of the immune response (innate and adaptive). Protozoan parasites including Leishmania, Toxoplasma, Plasmodium and Trypanosoma are able to change and affect host cell signalling and cellular mechanisms to their favour for developing pathogenicity in the infected host cells. The investigation of miRNAs as effective agents in regulating of such processes can help to understand more deeply the biology and pathogenicity of these parasites (Acuña et al., Reference Acuña, Floeter-Winter and Muxel2020; Paul et al., Reference Paul, Ruiz-Manriquez, Serrano-Cano, Estrada-Meza, Solorio-Diaz and Srivastava2020).
It has been revealed that Plasmodium parasite up-regulates several host miRNAs that target proteins involved in immune response and down-regulates miRNAs that contribute to the inhibition of parasitic translation, host cell proliferation, metabolism and survival (Paroo et al., Reference Paroo, Ye, Chen and Liu2009; Lourembam et al., Reference Lourembam, Sawian and Baruah2013). Toxoplasma parasite features its own miRNA processing system and is able to secret exosomes that contain miRNAs (Menard et al., Reference Menard, Haskins and Denkers2019). The expression of miR-146a and/or miR-155 in infected host cells with Leishmania, Toxoplasma and Plasmodium parasites reveal common characteristics that are implicated in the subversion of the host immune response (Guerfali et al., Reference Guerfali, Laouini, Guizani-Tabbane, Ottones, Ben-Aissa, Benkahla, Manchon, Piquemal, Smandi and Mghirbi2008; Hentzschel et al., Reference Hentzschel, Hammerschmidt-Kamper, Börner, Heiss, Knapp, Sattler, Kaderali, Castoldi, Bindman and Malato2014; Frank et al., Reference Frank, Marcu, Petersen, Weber, Stigloher, Mottram, Scholz and Schurigt2015; Acuña et al., Reference Acuña, Floeter-Winter and Muxel2020). By detecting the higher parasite burden in the liver and spleen of Leishmania donovani-infected miR-155 knockout mice, it was confirmed the effect of this miRNA on the host immune response in VL infection. Leishmania antigen-stimulated splenocytes from miR-155 knockout mice produced lower levels of T helper cell 1 (Th1)-associated interferon gamma (IFNγ) compared to controls (Varikuti et al., Reference Varikuti, Natarajan, Volpedo, Singh, Hamza, Messick, Guerau-de-Arellano, Papenfuss, Oghumu and Satoskar2019). A broad view regarding the role of miRNAs in protozoan parasites infections and the interaction with host cells has been briefly reviewed in recent years (Acuña et al., Reference Acuña, Floeter-Winter and Muxel2020; Paul et al., Reference Paul, Ruiz-Manriquez, Serrano-Cano, Estrada-Meza, Solorio-Diaz and Srivastava2020). In this sense, Table 1 summarized the critical role of miRNAs in some parasitic diseases and their pathological impact or clinical application.
Table 1. miRNAs in parasites and parasitic diseases and host-parasites interactions

In the current study, we have focused on the role of miRNAs expressed in Leishmania parasites and by their host cells that can explain the immunobiology of subversion, pathogenicity, survival, replication, drug resistance and treatment of these parasites.
miRNAs expressed in Leishmania parasites
The identification and characterization of miRNAs in Leishmania parasites and their plausible biological functions can facilitate the discovery of potential therapeutic targets in leishmaniasis. Some computational strategies have suggested that the target genes of several miRNA-like elements expressed in L. major were related to the multidrug resistant protein such as adenosine triphosphate (ATP) binding cassette (ABC) transporter and also ribosomal protein, hydrolase and exonuclease and RNA binding proteins (Chandra Sahoo et al., Reference Chandra Sahoo, Yousuf Ansari, Ranjan Dikhit, Gupta, Rana and Das2013).
The antiproliferative and apoptotic effect of trans-dibenzalacetone (DBA, a synthetic monoketone analogue of curcumin) on L. donovani has been previously reported (Chauhan et al., Reference Chauhan, Rao, Shankar, Chauhan, Kapadia and Singh2018), and several miRNAs including hsa-miR-151a, hsa-miR-15b and hsa-miR-30c-1 were identified as down-regulated markers in DBA treated intracellular amastigotes in comparison with untreated parasites (Singh and Chauhan, Reference Singh and Chauhan2018). On the other hand, miR-15b targets B-cell lymphoma 2 (Bcl-2) and the caspase signalling promoting apoptosis (Guo et al., Reference Guo, Pan, Li, Sun and Liu2009). Additionally, autophagy-related protein 5 (ATG5), as a target gene of miR-15b, is required for ATG8 dependant autophagy and phospholipid balance in the mitochondrion in L. major (Williams et al., Reference Williams, Smith, Cull, Mottram and Coombs2012).
miR-151a plays a role in the regulation of cellular respiration and ATP production by targeting cytochrome b. The downregulation of miR-151a, after DBA parasites treatment, induced mitochondrial dysfunction in Leishmania parasites (Zhou et al., Reference Zhou, Wang, Qin, Ji, Xu, Wu, Chen, Wu, Song and Shen2015; Singh and Chauhan, Reference Singh and Chauhan2018). miR-30a-3p is overexpressed in Leishmania infected cells (Singh et al., Reference Singh, Pandey, Shaha and Madhubala2016), however, it is downregulated in DBA-treated parasites, suggesting that the down-regulation of this miRNA could inhibit the replication and virulence of Leishmania parasites (Singh and Chauhan, Reference Singh and Chauhan2018).
The activity of ATG4 (autophagy-related proteins) is required for parasite viability, and it has been identified as a target of miR-30c (Williams et al., Reference Williams, Woods, Juliano, Mottram and Coombs2009, Reference Williams, Mottram and Coombs2013; Singh and Chauhan, Reference Singh and Chauhan2018). The down-regulation of ATG4 inhibits the cell viability of Leishmania parasites through the regulation of miR-30c expression. Summing up, DBA plays a major role in parasite survival and replication by affecting the expression of specific miRNAs which regulate the balance between autophagy and apoptosis (Singh and Chauhan, Reference Singh and Chauhan2018).
miRNAs expressed in Leishmania-infected host cells, tissues and sera
Leishmania parasites lead to the subversion/modulation of the innate immune response and cellular metabolic pathways in the host cells. Many host gene expression and signalling pathways are targeted by these parasites to modify host defences including immune activation, oxidative damage, antigen presentation and apoptosis, leading to parasite survival and replication. However, the molecular mechanisms used by these parasites to subvert the immune response are not fully clarified. Therefore, miRNA could be one of the most important regulatory factors to manipulate the host cells after infection (Diotallevi et al., Reference Diotallevi, De Santi, Buffi, Ceccarelli, Vitale, Galluzzi and Magnani2018).
miRNAs play fundamental roles on macrophage activation, polarization, tissue infiltration and resolution of inflammation. They can balance between pro- and anti-inflammatory signalling, integrating stimulus from damage-associated molecular patterns (DAMPs), pathogen-associated molecular patterns (PAMPs) and inflammatory and anti-inflammatory cytokines such as transforming growth factor beta (TGF-β), IFNγ, glucocorticoids, interleukin 4 (IL-4) among others (Curtale et al., Reference Curtale, Rubino and Locati2019). For instance, miR-155 expression is significantly enhanced when macrophages are polarized to the M1 phenotype; whereas it was considerably reduced in M2-polarized macrophages (Cai et al., Reference Cai, Yin, Li, Zhu, Zhang, Zhang and Zen2012). miRNAs have been indicated as important players potentially participating in the modulation of the early phase as well as the resolution of inflammation (Curtale et al., Reference Curtale, Rubino and Locati2019). Accordingly, miRNAs profiling in Leishmania-infected macrophages could reveal valuable information regarding immune responses, pathogenicity, survival, diagnosis, treatments and other biology aspects of these parasites.
Expression patterns of miRNAs in peripheral blood mononuclear cells (PBMCs) and macrophages
The differential expression pattern of miRNAs as well as their relationship with the immune response and parasite load have been recently investigated in PBMCs and splenic leucocytes (SL) of Canine VL (CVL)-infected dogs by L. infantum (Bragato et al., Reference Bragato, Melo, Venturin, Rebech, Garcia, Lopes and de Lima2018a, Reference Bragato, Melo, Venturin, Rebech, Garcia, Lopes and de Lima2018b; Melo et al., Reference Melo, Bragato, Venturin, Rebech, Costa, Garcia, Lopes, Eugênio, Patto dos Santos and de Lima2019). In infected PBMCs, miR-21, miR-194, miR-424 and miR-451 showed a three-fold expression increase, miR-192, miR-371 and miR-503 denoted two-fold increase in their expression, whereas a two-fold decrease in miR expression level was detected for miR-150 and miR-574. The parasite load in PBMCs was correlated to the differentially expressed miRNAs, supporting the strong positive correlation with the expression of miR-194, a positive correlation with miR-371 expression, and a negative correlation with miR-150 expression in PBMCs (Bragato et al., Reference Bragato, Melo, Venturin, Rebech, Garcia, Lopes and de Lima2018b). The increase level of miR-194 could be a mechanism to regulate the secretion of inflammatory cytokines, such as tumour necrosis factor alpha (TNF-α), modulating Leishmania parasite burden in infected animals (Bragato et al., Reference Bragato, Melo, Venturin, Rebech, Garcia, Lopes and de Lima2018b). Interestingly, miR-194 also showed a strong positive correlation with serum urea of CVL infected dogs, suggesting that miR-194 could be useful as a possible early plasma biomarker in renal lesion of dogs infected with CVL (Wang et al., Reference Wang, Yi-feng, Li, Wang, Bian, Lai and Yu2014; Esch et al., Reference Esch, Schaut, Lamb, Clay, Lima, Do Nascimento, Whitley, Jeronimo, Sutterwala and Haynes2015). The expression of miR-371 was also increased in infected-PBMCs and showed a positive correlation with parasite load in PBMCs, suggesting that this miR could be associated with permissive immune response in CVL. Furthermore, miR-194 represented a potential negative correlation with haemoglobin concentration and miR-371 illustrated a strong negative correlation with erythrocyte globular volume (Bragato et al., Reference Bragato, Melo, Venturin, Rebech, Garcia, Lopes and de Lima2018b). miR-150 as a detected down-regulated miRNA exhibited a negative correlation with Leishmania parasitic load in the blood (Zhou et al., Reference Zhou, Wang, Mayr, Bartel and Lodish2007). miR-150 is probably acting in hypergammaglobulin and also in the development of regulatory B-cells, by enhancing the Leishmania parasite load due to T-cell suppression in CVL. On the other hand, the reduction in Natural Killer (NK) cells, modulated by miR-150, could be associated with the higher parasite burden in the PBMC of CVL-infected animals (Bragato et al., Reference Bragato, Melo, Venturin, Rebech, Garcia, Lopes and de Lima2018b).
Similarly, microarray analyses indicated the enhanced expression level of miR-7, miR-21, miR-148a and miR-615, and the downregulation of miR-125a, miR-125b and miR-150 in infected-SL compared to control leucocytes. miR-148a targets genes involved in the regulation of apoptosis such as FAS and FAS ligand (FASLG) suggesting the role of this miRNA in the death of CD4+ and CD8+ cells in CVL-infected dogs (Melo et al., Reference Melo, Bragato, Venturin, Rebech, Costa, Garcia, Lopes, Eugênio, Patto dos Santos and de Lima2019). miR-615 targets ligand-dependant nuclear corepressor (LCoR), a derepressor of peroxisome proliferator-activated receptor gamma (PPARγ), which increases the phagocytic capacity of splenic macrophages (Jiang et al., Reference Jiang, Zhang, Li, Liang, Ren, Li, Pu and Yang2011). miR-21 as another up-regulated miRNA probably contributes in the reduction of TNF-α level, which could lead to the increase of splenic parasite load and disease progression (Alves et al., Reference Alves, de Amorim, Moura, Ribeiro, Alves, Michalick, Kalapothakis, Bruna-Romero, Tafuri and Teixeira2009; Carissimi et al., Reference Carissimi, Carucci, Colombo, Piconese, Azzalin, Cipolletta, Citarella, Barnaba, Macino and Fulci2014; Mazloom et al., Reference Mazloom, Alizadeh, Esfahani, Razi and Meshkani2016). As well known, IL-12 is an essential cytokine for activation of NK cells and IFN-γ production by T cells and polarization of immune response to Th1 during CVL (Strauss-Ayali et al., Reference Strauss-Ayali, Baneth, Shor, Okano and Jaffe2005). The transfection of infected-SL with a miR-21 inhibitor led to the increase of IL-12 cytokine and the T-box expressed in T cells (T-bet)/GATA-binding protein 3 (GATA-3) ratio (increasing Th1 profile population), and reduced Leishmania parasite load in infected-SL and revealed the interesting role of miR-21 in the inhibition of IL-12. These data highlighted that L. infantum infection changed the expression of miRNAs in L. infantum infected-PBMCs and -SL and that miRNAs including miR-21, miR-194, miR-371 and miR-150 interfered in the cellular immune response of L. infantum-infected dogs and also suggested such miRNAs as a plausible therapeutic target in CVL (Melo et al., Reference Melo, Bragato, Venturin, Rebech, Costa, Garcia, Lopes, Eugênio, Patto dos Santos and de Lima2019).
Evidences have shown that within the first 24 h of L. major infection of human primary macrophages induce a rapid change in the host miRNA profile. Alterations in the levels of miR-22, miR-133b, miR-155 and miR-210 have been associated with the host cell responses to apoptosis (Cheng et al., Reference Cheng, Byrom, Shelton and Ford2005; Lemaire et al., Reference Lemaire, Mkannez, Guerfali, Gustin, Attia, Sghaier, Dellagi, Laouini and Renard2013). The expression level of miR-210 is significantly increased from 6 h to 24 h after L. major infection of macrophages. After silencing miR-210, the caspase-3 activity (as an apoptotic indicator) increased in HeLa cells (Cheng et al., Reference Cheng, Byrom, Shelton and Ford2005). Therefore, miR-210 up-regulation in Leishmania-infected macrophages might participate in the anti-apoptotic response of infected macrophages via caspase-3 inhibition.
In the same way, L. major infection induced the expression level of miR-24-3p as an anti-apoptotic factor in the first hours of infection in favour of its survival. miR-24-3p can interact and regulate caspase 3 gene to expand the life time of macrophage and establish the parasite infection (Lasjerdi et al., Reference Lasjerdi, Ghanbarian, Yeganeh, Tabaei, Mohebali, Taghipour, Koochaki, Hamidi, Gholamrezaei and Haghighi2020). Accordingly, the use of an antagomir-24-3p might be a possible therapeutic strategy for L. major treatment.
Additionally, the use of miR-15a mimic, miR-155 inhibitor or both of them increases the apoptosis rate of infected macrophages in vitro, and reduces the size of lesions in vivo within 6 weeks after the infection (Gholamrezaei et al., Reference Gholamrezaei, Rouhani, Mohebali, Mohammadi-Yeganeh, Hoseini, Haghighi, Lasjerdi, Hamidi and Sharifi-Yazdi2020) suggesting that miRNA-based therapy could be a possible novel treatment for cutaneous leishmaniasis.
The let-7 miRNA family is conserved from parasites to humans and correlated with the acute innate immune response, cell differentiation, development and therapeutic strategies by targeting caspase-3 (Lee et al., Reference Lee, Kim, Chung, Kim and Dutta2005; Boyerinas et al., Reference Boyerinas, Park, Hau, Murmann and Peter2010). Let-7a is also able to induce cell apoptosis and cell cycle arrest (Zhao et al., Reference Zhao, Hu, Hao, Zhang, Guo, Li, Xie, Liu, Wang and Zhang2018). The increased level of let-7a probably manipulate host cells in order to alter miRNA levels and regulate macrophage functions during infection (Hashemi et al., Reference Hashemi, Sharifi, Tolouei, Hashemi, Hashemi and Hejazi2018b). Inhibiting let-7a by using a locked nucleic acid (LNA) oligonucleotide (Ørom et al., Reference Ørom, Kauppinen and Lund2006) increased the apoptotic and necrotic process of L. major-infected human monocyte-derived macrophages in-vitro (Hashemi et al., Reference Hashemi, Sharifi, Masjedi, Tolouei, Hashemi, Mortazavidehkordi, Mohaghegh, Hashemi and Hejazi2018a). Since apoptosis suppression is a strategy used by Leishmania parasites to evade the host immune response (Gupta et al., Reference Gupta, Srivastav, Saha, Das and Ukil2016), the inhibition of let-7a might revealed new insights for the treatment of leishmaniasis.
Unfolded protein response (UPR) (endoplasmic reticulum (ER) stress response) is an evolutionary conserved mechanism aimed to restore ER homeostasis and ensure cell survival (Schröder, Reference Schröder2008). L. infantum is able to induce UPR as a critical pathway to promote infection progression in macrophages (Dias-Teixeira et al., Reference Dias-Teixeira, Calegari-Silva, Santos, Santos, Lima, Medina, Aktas and Lopes2016; Galluzzi et al., Reference Galluzzi, Diotallevi, De Santi, Ceccarelli, Vitale, Brandi and Magnani2016). Different miRNAs have been shown to participate in UPR signalling (Maurel and Chevet, Reference Maurel and Chevet2013). Thus, the UPR-activated transcription factor sXBP1 is able to up-regulate the expression of miR-346 in L. infantum- and L. viannia-infected macrophages (U937 and THP-1). For example, RFX1, a miR-346 predicted target gene, was significantly down-regulated 48 h post-infection. Additionally, several major histocompatibility complex (MHC)- or interferon-associated genes were suggested as targets of miR-346, indicating a critical role of this miRNA on regulating macrophage functions and as an attractive druggable anti-Leishmania drug target (Diotallevi et al., Reference Diotallevi, De Santi, Buffi, Ceccarelli, Vitale, Galluzzi and Magnani2018).
TLR2 and TLR4 mediated L. amazonensis recognition and infectivity resistance in macrophages. In addition, myeloid differentiation primary response 88 (MYD88)-dependant receptors probably play a role in macrophage activation in response to L. amazonensis (Muxel et al., Reference Muxel, Acuña, Aoki, Zampieri and Floeter-Winter2018a). It has been shown that the knockout of TLR2, TLR4 and MYD88 genes changed the rate of expressed miRNAs modulated in murine bone marrow-derived macrophages infected by L. amazonensis, including the down-regulation of let-7e expression, and then increased the parasites burden in these cells compared to the control. let-7e regulates pro- and anti-inflammatory responses during infection or TLR/PAMP stimulation by inducing NF-κβ (nuclear factor kappa-light-chain-enhancer of activated B cells) activation and cytokine production. Based on these results, the expression of miRNAs including let-7e, let-7f and let-7g requires MYD88, TLR2 and TLR4 signalling during L. amazonensis infection, highlighting the role of TLR pathway in the transcriptional and post-transcriptional regulation of gene expression during Leishmania infection (Muxel et al., Reference Muxel, Acuña, Aoki, Zampieri and Floeter-Winter2018a). As abovementioned, TLR2, TLR4 and MYD88 exerted a regulatory function in miRNA expression, such as let-7e, during the course of infection. TLR2, TLR4 and MYD88 signalling changed the expression of genes involved in polyamine/nitric oxide (NO) production in L. amazonensis-infected macrophages. Let-7e affected L. amazonensis infectivity by regulating L-arginine metabolism. Leishmania parasites survival in macrophages depended on the deviation of L-arginine metabolism to the production of polyamines (Muxel et al., Reference Muxel, Aoki, Fernandes, Laranjeira-Silva, Zampieri, Acuña, Müller, Vanderlinde and Floeter-Winter2018b). Therefore, let-7e inhibition indirectly affected the expression of genes involved in L-arginine metabolism, increasing NO production and the subsequent parasite infectiveness (Muxel et al., Reference Muxel, Acuña, Aoki, Zampieri and Floeter-Winter2018a). There are several studies that have highlighted the differential expression of miRNAs in Leishmania-infected macrophages (Table 2).
Table 2. Differential expression of miRNAs in Leishmania-infected macrophages

The regulatory function of miRNAs on T cell subset in leishmaniasis
VL immunopathology is determined by mixed production of Th1/2 cytokines and the disease is fixed by an increased level of Th2 cytokine (Gupta et al., Reference Gupta, Oghumu and Satoskar2013). CD4+ T cells are main cell type responsible for the production of Th1/2 cytokine in the infected host cell by Leishmania parasites (Colpitts and Scott, Reference Colpitts and Scott2010). During human VL, the plasticity of T cell proliferation and differentiation is related to the miRNA-mediated gene regulation which balance the Th1/Th2 or Th17/regulatory T cells (Tregs) type of immune response (Li et al., Reference Li, Chau, Ebert, Sylvester, Min, Liu, Braich, Manoharan, Soutschek and Skare2007; Nakahama et al., Reference Nakahama, Hanieh, Nguyen, Chinen, Ripley, Millrine, Lee, Nyati, Dubey and Chowdhury2013). Th2 and Treg immune cells are critical in VL progression and Th1 and Th17 specific immune response are central to control this infectious disease. In this sense, miRNAs play important regulatory functions during the differentiation of naive CD4+ T and the balance among these specific skewed immune responses in Leishmania infection (Kumar et al., Reference Kumar, Das, Kumar, Tiwari, Kumar, Abhishek, Mandal, Kumar, Shafi and Bamra2020b). Accordingly, some relevant information was summarized in Table 3 highlighting the regulatory function of miRNAs on T cell subset in leishmaniasis. Such data further show that miRNAs through a regulatory function to control CD4+ T cell differentiation, have a potential capacity to regulate immune signalling, cytokine production and immune cell migration to manage and control the human VL (Pandey et al., Reference Pandey, Sundar and Prajapati2016).
Table 3. The regulatory function of miRNAs on T cell subset in leishmaniasis

miRNAs expressed in Leishmania-infected tissues and sera
Abnormal lipid profiles were reported in VL patients (Liberopoulos et al., Reference Liberopoulos, Apostolou, Gazi, Kostara, Bairaktari, Tselepis and Elisaf2014; Tsimihodimos et al., Reference Tsimihodimos, Kei, Apostolou and Elisaf2018), and had been observed in an animal VL infection model highlighting the fascinating association between the change of lipid metabolism (altered expression levels of lipid metabolic genes) and the liver miR-122 levels (Ghosh et al., Reference Ghosh, Bose, Roy and Bhattacharyya2013). miR-122 represents more than 70% of liver-miRNAs and is responsible for liver homoeostasis and lipid metabolism (fatty acid and cholesterol metabolism) (Elmen et al., Reference Elmen, Lindow, Silahtaroglu, Bak, Christensen, Lind-Thomsen, Hedtjärn, Hansen, Hansen and Straarup2008; Girard et al., Reference Girard, Jacquemin, Munnich, Lyonnet and Henrion-Caude2008). RNase III endonuclease Dicer1 is able to process the change of pre-miRNAs to the mature form in the cytoplasm (Filipowicz et al., Reference Filipowicz, Bhattacharyya and Sonenberg2008). It has been indicated that leishmanial-metalloprotease glycoprotein 63 (gp63), a Zn-metalloprotease, targets Dicer1 inducing a decrease of miR-122 activity in human hepatic cells, as well as in L. donovani-infected mouse liver (Ghosh et al., Reference Ghosh, Bose, Roy and Bhattacharyya2013). This strategy also clarified the adaptation of parasites to combat regulatory RNA functions in host cells. Interestingly, the restoration of miR-122 or Dicer1 levels in VL mouse liver enhanced serum cholesterol and decreased liver parasite burden and survival (Ghosh et al., Reference Ghosh, Bose, Roy and Bhattacharyya2013). These results illustrated the strategies used by Leishmania parasites to control liver miR-122 and to modulate serum cholesterol.
The miRNA expression might change during pathological processes like localized cutaneous leishmaniasis (LCL) induced by L. braziliensis. It has been demonstrated that the expression of miR-193b and miR-671 are greatly associated with their target genes, CD40 and TNF receptor (TNFR), underlining the critical function of these miRNAs in the expression of genes correlated to the inflammatory response in LCL. Interestingly, miR-193b and miR-671 correlate in patients who had faster wound healing (<59 days) but not in patients who need longer cure period (>60 days). Due to the association of such miRNAs with the control of inflammation and the healing time of LCL, they can be suggested as possible predictive markers of prognosis (Nunes et al., Reference Nunes, Silva, Ampuero, Noronha, Souza, Correia, Khouri, Boaventura, Barral and Ramos2018).
The inflammasome, which is induced during Leishmania infection, involves the activation of caspase-1 and the release of the proinflammatory cytokines IL-1β and IL-18 that promote an inflammatory response and pyroptosis by triggering the release of more cytokines, the activation of other immune cells and programmed cell death (Di Virgilio, Reference Di Virgilio2013). It was suggested that miRNAs exert a modulatory function in the assembly of the inflammasome complex (Nokoff and Rewers, Reference Nokoff and Rewers2013). The analysis of serum cytokines and the expression of circulating miRNAs in patients with CL showed increased levels of miR-7-5p, miR-133a, miR-146b, miR-223-3p and miR-328-3p, associated with the high levels of IL-1β, IL-6 and IL-17 compared to controls (Mendonça et al., Reference Mendonça, Santos, Kaneto, de Carvalho, Lima-Santos, Augusto, Carvalho, Soares-Martins and Silva-Jardim2020). These cytokine profiles in patients with CL may be triggering a Th17 immune response and enhancing IL-1β levels and inflammasomes activation. The overexpressed miRNAs profile in those patients is associate with the transcriptional control of several immune response genes, such as those involved in the regulation of programmed cell death (DNAJB6, DNAJC5, IRS2, RBPJ, IGF1R, ECT2, MEF2C, FOXO3, FOXO1 and TGFB2), caspase activity (NLRP3, SENP1, FOXL2, F3 and SNCA) and response to cytokine stimuli (IRAK1, IL-6ST, TRAF6, MCL1 and BCL2L1). Data analysis showed an inverse correlation between the levels of IL-1β and the miR-7 and miR-223 in CL patients, whereas the levels of miR-133a, miR-146b and miR-328 showed positive values compared to IL-1β levels. These results indicated that miR-7, miR-133a and miR-223 played a critical role in the inflammasome activation (Mendonça et al., Reference Mendonça, Santos, Kaneto, de Carvalho, Lima-Santos, Augusto, Carvalho, Soares-Martins and Silva-Jardim2020). This information is very important to better understand the interplay between miRNAs and cytokines during CL infection.
The higher levels of serum exosomal miR-122 was recently identified as a good biomarker for liver diseases in leishmaniotic dogs. This result suggested that alterations of the lipid metabolism, low HDL (high-density lipoprotein) and high LDL (low-density lipoprotein) serum levels along with a lower miR-122 expression indicate a hepatic alteration induced by L. infantum in dogs (Loria et al., Reference Loria, Dattilo, Santoro, Guccione, De Luca, Ciaramella, Pirozzi and Iaccino2020). However, more investigations are needed to better define the role of miR-122 as a potential biomarker of hepatic damage/dysfunction during canine leishmaniasis.
Differential expression of miRNAs associated with Leishmania survival, parasite burden, replication and infectivity
It has been revealed that Leishmania is able to reside successfully in the macrophages phagolysosomes, developing the parasitophorous vacuole (PV) that contains lysosomal markers including cathepsin D, lysosome associated membrane protein 1 (Lamp1) and Lamp2 (McConville et al., Reference McConville, De Souza, Saunders, Likic and Naderer2007). Accordingly, the Rab GTPases, involved in endosomal biogenesis, are considered potential targets of intracellular pathogens to subvert immune response (Spanò and Galán, Reference Spanò and Galán2018). L. donovani upregulates the expression of Rab5a (an early endosomal protein) in infected THP-1 macrophages by downregulating the expression level of miR-494. Subsequently, Leishmania parasites recruit and maintain Rab5a and early endosome associated antigen 1 (EEA1) on the PV allowing the parasites to reside in the early endosomal compartment without fusing with the lysosomes. The inhibition of the expression of Rab5a by promoting miR-494 expression or the knock down of Rab5a gene by siRNA will probably lead the internalized parasites endosome to the lysosomes fusion reducing the parasite survival and evasion (Verma et al., Reference Verma, Rastogi and Mukhopadhyay2017). This information highlighted the essential role of miR-494 and Rab5a, for the survival of Leishmania parasites in human macrophages.
The analysis of miRNA profiling in L. amazonensis-infected macrophage showed that the lack of L. amazonensis arginase (La-arg −) led to distinct regulation of miRNA expression profiles in infected macrophages (Muxel et al., Reference Muxel, Laranjeira-Silva, Zampieri and Floeter-Winter2017). Seventy-eight percentage of altered miRNAs were upregulated in macrophages infected with La-WT parasites, whereas only 32% were up-regulated in macrophages infected with La-arg −. The lack of L. amazonensis arginase (La-arg −) inhibited the expression of two macrophage miRNAs, miR-294 and miR-721, which are involved in the interaction and regulation of nitric oxide synthase 2 (NOS2) and NO production. The absence of these miRNAs led to the reduction of parasite infectivity by promoting the NO production and suggesting NOS2 as a target of the aforementioned miRNAs. Leishmania can use the parasite arginase/L-arginine metabolism to subvert NO production in macrophage, by inducing miR-294 and miR-721 (Muxel et al., Reference Muxel, Laranjeira-Silva, Zampieri and Floeter-Winter2017). Summarizing, these miRNAs could be pointed out as new targets for drug development.
Some strains of the L. guyanensis harbour a viral endosymbiont known as Leishmania RNA virus 1 (LRV1) (Ives et al., Reference Ives, Ronet, Prevel, Ruzzante, Fuertes-Marraco, Schutz, Zangger, Revaz-Breton, Lye and Hickerson2011) and TLR-3 recognition of these LRV1s increased Leishmania parasite burden and lesion swelling (Eren et al., Reference Eren, Reverte, Rossi, Hartley, Castiglioni, Prevel, Martin, Desponds, Lye and Drexler2016). However, the relationship between anti-viral innate immune responses and parasitic infection remains unknown. It seems that miR-155 is upregulated in macrophages infected with LRV1+ L. guyanensis in comparison with LRV1− strain. The LRV1-driven miR-155 expression was dependant on TLR-3/TIR-domain-containing adaptor-inducing IFN-β (TRIF) signalling. This activation pathway increased parasite persistence by enhancing macrophage survival. Interestingly, phosphatidylinositol 3-kinase (PI3K)/protein kinase B (AKT) (PI3K/AKT) inhibition led to the reduction of LRV1-mediated macrophage survival as well as parasite burden. Moreover, miR-155-deficient mice significantly decrease the LRV1-induced disease severity and the Akt phosphorylation in macrophages obtained from the infected mice (Eren et al., Reference Eren, Reverte, Rossi, Hartley, Castiglioni, Prevel, Martin, Desponds, Lye and Drexler2016).
L. donovani led to the overexpression of miR-210 and hypoxia-inducible factor-1α (HIF-1α) in the host macrophages (Kumar et al., Reference Kumar, Kumar, Das, Kumar, Abhishek, Verma, Mandal, Singh and Das2018) via a hypoxia-independent pathway (Chan et al., Reference Chan, Banerjee, Choi and Sen2012; Singh et al., Reference Singh, Mukhopadhyay, Biswas, Singh and Mukhopadhyay2012). The miR-210 expression was transcriptionally controlled by HIF-1α and was dependant on Leishmania-induced HIF-1α activation (Lemaire et al., Reference Lemaire, Mkannez, Guerfali, Gustin, Attia, Sghaier, Dellagi, Laouini and Renard2013; Kumar et al., Reference Kumar, Kumar, Das, Kumar, Abhishek, Verma, Mandal, Singh and Das2018). Furthermore, macrophages infected with L. donovani and treated with siRNA for HIF-1α or antagomir-210 significantly reduced the parasitic burden and infectivity rate (Kumar et al., Reference Kumar, Kumar, Das, Kumar, Abhishek, Verma, Mandal, Singh and Das2018). The upregulated miR-210 inhibited TNF-α receptor family leading to reduce the synthesis of different pro-inflammatory cytokines, which facilitated the parasite survival inside the macrophages. After silencing miR-210 with antagomir, pro-inflammatory cytokines genes such as TNF-α and IL-12 were increased in miR-210 inhibited macrophages. This process also further promoted and increased the Reactive Oxygen Species (ROS) and NO production inducing the elimination of Leishmania parasites in infected macrophages (Kumar et al., Reference Kumar, Kumar, Das, Kumar, Abhishek, Verma, Mandal, Singh and Das2018).
Interestingly, Leishmania infection was able to significantly up-regulate the expression level of host c-Myc inducing miRNA suppression. Indeed, c-Myc silencing decreased the intracellular survival of parasite suggesting that c-Myc is required for the pathogenicity of Leishmania (Colineau et al., Reference Colineau, Lambertz, Fornes, Wasserman and Reiner2018). Accordingly, c-Myc inhibitors can be considered as a possible therapeutic target for leishmaniasis (Whitfield et al., Reference Whitfield, Beaulieu and Soucek2017).
Melatonin, the darkness-signalling hormone, plays a critical role in the modulation of macrophage activation and controlling the inflammatory response during parasitic infection (Markus et al., Reference Markus, Fernandes, Kinker, da Silveira Cruz-Machado and Marçola2018; Xia et al., Reference Xia, Chen, Zeng, Zhao, Zhu, Deng, Zhu, Yin, Wang and Hardeland2019). Recently, exogenous melatonin treatment of BALB/c macrophages was found to decrease L. amazonensis parasite burden and modulated host miRNAs expression profile (miR-294-3p, miR-302d-3p and miR-30e-5p) (Fernandes et al., Reference Fernandes, Aoki, Maia Acuña, Zampieri, Markus, Floeter-Winter and Muxel2019). Melatonin treatment also decreased IL-6, monocyte chemoattractant protein-1 (MCP-1), RANTES (Regulated upon Activation, Normal T Cell Expressed and Presumably Secreted) and macrophage inflammatory protein-2 (MIP-2), as well as IL-10 levels in infected macrophages (Lebovic et al., Reference Lebovic, Chao, Martini and Taylor2001; Marçola et al., Reference Marçola, da Silveira Cruz-Machado, Fernandes, Monteiro, Markus and Tamura2013; Fernandes et al., Reference Fernandes, Aoki, Maia Acuña, Zampieri, Markus, Floeter-Winter and Muxel2019). miR-294-3p targets NOS2 mRNA decreasing NOS2 expression and promoting infectivity (Muxel et al., Reference Muxel, Laranjeira-Silva, Zampieri and Floeter-Winter2017) and its inhibition drives high expressions of TNF and Mcp-1/chemokine ligand 2 (Ccl2) that reduce infectivity. In addition, miR-302d has also been described as a regulator of NOS2 expression (Farlik et al., Reference Farlik, Reutterer, Schindler, Greten, Vogl, Müller and Decker2010; Smith et al., Reference Smith, Fernando, Wu, Seo, Gabhann, Piskareva, McCarthy, Howard, O'Connell and Conway2017), and melatonin treatment or miR-302d-3p or miR-30e-5p inhibition enhanced NOS2 mRNA expression and NO production, decreasing macrophages infection. In fact, melatonin treatment of Leishmania-infected macrophages changes the balance of L-arginine metabolism by inducing NOS2 in detriment of arginase 1 (Arg1) and thus altering infectivity (Fernandes et al., Reference Fernandes, Aoki, Maia Acuña, Zampieri, Markus, Floeter-Winter and Muxel2019).
IL-12 produced by dendritic cells (DCs) is essential for starting a host protective Th1 cell response, but miR-21 has been indicated as a key negative regulatory factor of the expression of IL-12 mRNA during leishmaniasis infection. High levels of miR-21 were associated with low expressions of IL-12 mRNA in DCs infected with virulent Leishmania strains. Furthermore, silencing miR-21 enhances the IL-12 expression in DCs, during the infection with a virulent strain. These results suggest the critical role of miR-21 in mediating suppression of this cytokine. The infection of DCs with attenuated strains of Leishmania and suggesting that the levels of miR-21 could be measured as anti-leishmanial response of vaccines. Accordingly, lower levels of miR-21 could represent better immunogenicity and protective immune response of the vaccine (Bhattacharya et al., Reference Bhattacharya, Ismail, Kaul, Gannavaram and Nakhasi2017; Gannavaram et al., Reference Gannavaram, Bhattacharya, Siddiqui, Ismail, Madhavan and Nakhasi2019). Figure 3 has summarized several miRNAs expressed in Leishmania-infected host cells that could be involved in the survival, replication and infectivity of Leishmania parasites.
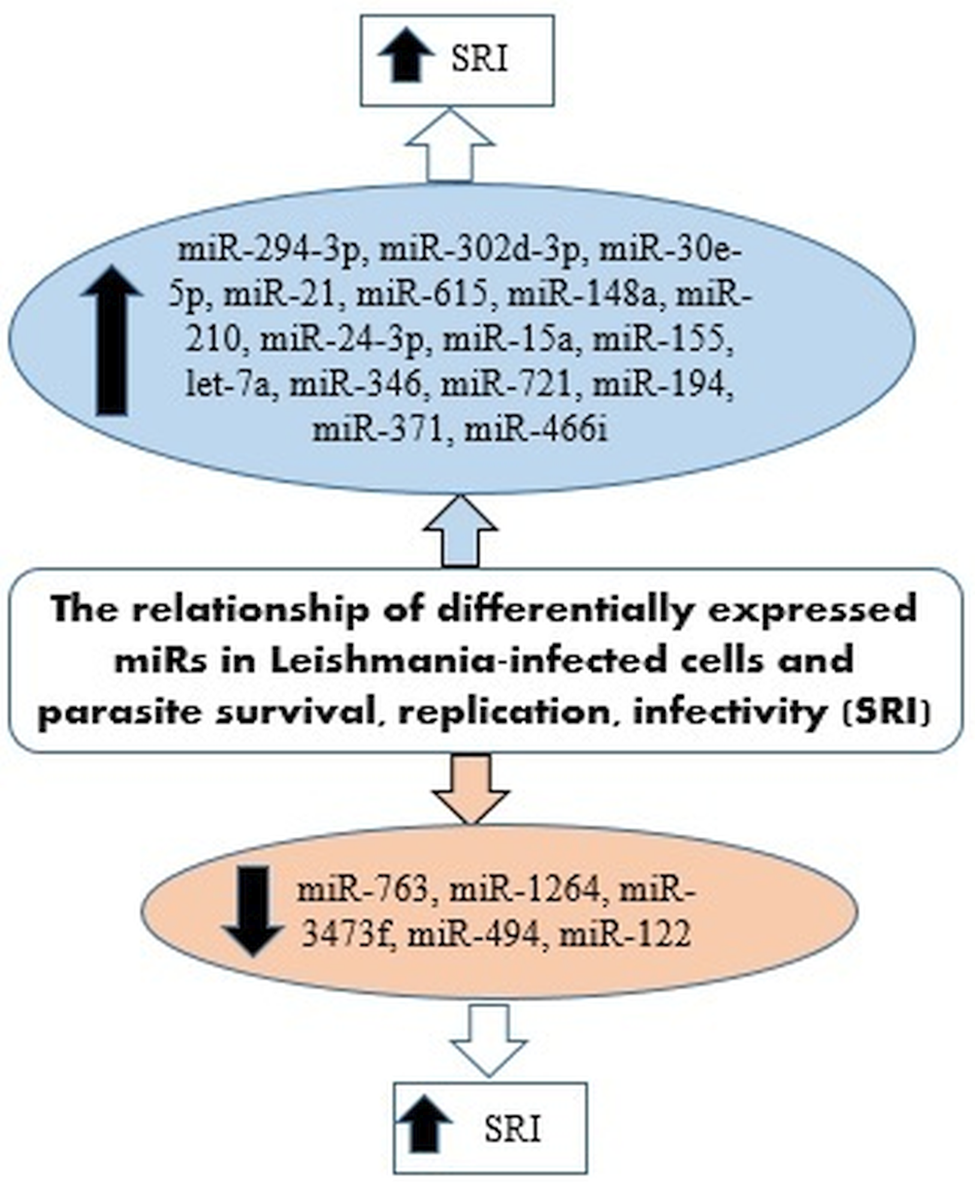
Fig. 3. miRNAs expressed in Leishmania-infected host cells involved in the survival, replication and infectivity of the parasite.
Expression of miRNAs in host cells and parasite drug resistance
miRNAs play an important role in drug resistance by altering the drug transporters, receptors and ion channels, thus, reducing the sensitivity of drugs (To, Reference To2013; Ren et al., Reference Ren, Gao, Sun, Zhang, Wang, Hua, Wan and Li2015; Nawaz et al., Reference Nawaz, Malik, Hameed and Zhou2019). The identification of miRNAs related to Leishmania parasites drug resistant could provide mechanistic details to combat drug resistance in leishmaniasis. Some studies revealed that Leishmania parasites induced the up-regulation of ABC transporters in macrophages by down regulating miR-763, miR-1264 and miR-3473f generating the efflux out of drugs (Singh et al., Reference Singh, Mishra, Bajpai, Singh and Tiwari2014; Tiwari et al., Reference Tiwari, Kumar, Gedda, Singh, Singh, Singh and Singh2017).
Infections of mammals with L. donovani resistant (LDR) lead to aggressive pathologies as compared to their sensitive strains (LDS) coupled with higher levels of IL-10 and TGF-β. The IL-10 increases the upregulation of multidrug- resistant protein-1 which produces the efflux of antimonials drugs from LDR infected-host cells (a key mechanism of antimony resistance) (Guha et al., Reference Guha, Das, Ghosh, Sundar, Dujardin and Roy2014). Considering that miRNAs are involved in the control of cytokines expression (Garavelli et al., Reference Garavelli, De Rosa and de Candia2018), the alteration of miRNA profile in the host cell could be an effective strategy to ensure infection or drug resistance by pathogens. Accordingly, targeting miRNA pathway might be a novel strategy to control infection caused by pathogens such as LDR parasites (Mukherjee et al., Reference Mukherjee, Mukherjee, Nanda, Mukhopadhayay, Ravichandiran, Bhattacharyya and Roy2020). The clinical manifestations of L. donovani infection are related to the critical balance of pro- and anti-inflammatory cytokines which is obtained through the miRNA-mediated regulation and by targeting the miRNA modulators, HuR and protein phosphatase 2A (PP2A) (Goswami et al., Reference Goswami, Mukherjee, Mazumder, Ganguly, Mukherjee, Chakrabarti, Roy, Sundar, Chattopadhyay and Bhattacharyya2020). Argonaute 2 (Ago2) phosphorylation may impair the binding of the protein with miRNAs and to the corresponding target mRNAs, therefore, the dephosphorylated form of Ago2 is required for miRNA activity (Chakrabarty and Bhattacharyya, Reference Chakrabarty and Bhattacharyya2017). On the other hand, phosphorylation and de-phosphorylation of Ago2 is controlled by PP2A and HuR. HuR is a miRNA derepressor protein and a miRNA sponge for specific miRNAs to negate their action on target mRNAs. HuR acts as a balancing factor of immune responses to disrupt the macrophage infection by the protozoan parasite. Leishmania parasites target HuR to promote the initiation of anti-inflammatory responses in infected macrophages. These parasites also induce the overexpression of PP2A that maintain Ago2 in dephosphorylated form, causing strong repression on the miRNA-targeted pro-inflammatory cytokines to promote an anti-inflammatory response in infected macrophages. HuR has an inhibitory effect on PP2A expression, and evidence suggested antagonistic miRNA-modulatory functions of HuR and PP2A which mutually balances immune response in macrophage by targeting miRNA function. Consequently, the expression of HuR and the simultaneous inhibition of PP2A can induce strong pro-inflammatory responses in the host macrophage to prevent the virulent antimonial drug sensitive or drug-resistant form of L. donovani infection (Goswami et al., Reference Goswami, Mukherjee, Mazumder, Ganguly, Mukherjee, Chakrabarti, Roy, Sundar, Chattopadhyay and Bhattacharyya2020). LDS and LDR upregulate PP2A and downregulate HuR at various levels inducing different levels from anti-inflammatory to proinflammatory cytokine production and generating disease manifestations in the host. HuR expression alone is sufficient to remove LDS infection, however, simultaneous increasing levels of HuR and inhibition of PP2A are needed to inhibit LDR mediated infection (Mukherjee et al., Reference Mukherjee, Mukherjee, Nanda, Mukhopadhayay, Ravichandiran, Bhattacharyya and Roy2020). Moreover, the analysis of predicted miRNAs with related binding sites in host cytokine transcripts identified a maximum number of interactions with IFN-γ transcript suggesting a possible and unknown function of IFN-γ in LDR infection. Among other Th1 cytokines IFN-α, IFN-β, IL-12, TNF-α and IL-6 also represented significant amount of interactions. The major Th2 cytokines with probable miRNA binding sites contain IL-10 and TGF-β while Th-17 cytokines like IL-17 and IL-27 also showed considerable number of potential interaction sites. Among the identified miRNAs, miR-487b, miR-669d, miR-669a-5p, miR-1251, miR-1381-1 and miR-2139 showed minimum number of interactions (Mukherjee et al., Reference Mukherjee, Mukherjee, Nanda, Mukhopadhayay, Ravichandiran, Bhattacharyya and Roy2020).
Antimony-resistant L. donovani (SbRLD) parasites interact with TLR2/TLR6 to induce IL-10 by exploiting p50/c-Rel subunits of NF-kB in infected macrophages (Mukherjee et al., Reference Mukherjee, Mukhopadhyay, Bannerjee, Chowdhury, Mukherjee, Naskar, Allam, Chakravortty, Sundar and Dujardin2013). Most of the TLRs can exploit the universal adaptor protein MYD88 to activate the transcription factor NF-κβ (Jefferies et al., Reference Jefferies, Bowie, Brady, Cooke, Li and O'Neill2001). It has been indicated that infections of macrophages from MYD88−/− mice with SbRLD significantly enhance the intracellular Leishmania parasite number coupled with the increased IL-10/IL-12 ratio in the culture supernatant in comparison with infections of wild type (WT) macrophages. In contrast, the infection with SbSLD cannot induce such a process. Infections of MYD88−/− macrophages or IL-12−/− macrophages with SbRLD induced high levels of IL-10 at 4 h, whereas the level of the same cytokine was increased after 12 h in WT macrophages, indicating that the absence of IL-12 favoured early binding of NF-κβ subunits to the IL-10 promoter, leading to the increase of IL-10 levels. MYD88 signalling is critical in maintaining IL-12 levels, but the up-regulation of miR-466i after SbRLD infection lead to the degradation of MYD88 and subsequently a reduction in IL-12 levels (Mukherjee et al., Reference Mukherjee, Mukherjee, Mukhopadhyay, Naskar, Sundar, Dujardin and Roy2014, Reference Mukherjee, Paul, Mukherjee, Mukhopadhyay, Das, Naskar, Sundar, Dujardin, Saha and Roy2015). Consequently, the reduced levels of IL-12 activate IL-10 promoter resulting an IL-10 increase in the host. Therefore, SbRLD use a significant strategy to evade host anti-leishmanial immune responses by manipulating host MYD88 to its favour (Mukherjee et al., Reference Mukherjee, Paul, Mukherjee, Mukhopadhyay, Das, Naskar, Sundar, Dujardin, Saha and Roy2015). Thus, the selection of approaches to restore MYD88 signalling by targeting miR-466i might be an attractive tool in managing SbRLD parasite-mediated leishmaniasis.
Conclusions and future directions
The identification of parasite miRNAs and those induced in the host cell by the infection brought new insights and understanding regarding the pathogenesis and druggable targets against parasitic diseases such as Leishmania infections. For instance, high levels of miRNAs in a specific tissue or serum of infected animals suggested them as possible biomarkers for that disease. Despite of standardized protocols for the current clinical practise, miRNAs screening constitutes a reliable tool for future use. Further investigations will bring more criteria needed to be used as appropriate biomarkers, including accessibility, high specificity and sensitivity (Condrat et al., Reference Condrat, Thompson, Barbu, Bugnar, Boboc, Cretoiu, Suciu, Cretoiu and Voinea2020). Moreover, this review intends to put together, most relevant information regarding Leishmania-specific miRNAs and their targets in hosts cells, as well as the mechanisms used by miRNAs to interfere with host pathophysiology of leishmaniasis at the molecular level. The investigation of exosomes and their miRNA contents will be very helpful for future chemotherapies and vaccination. These studies tried to identify, unique or highly different miRNA molecules as possible druggable targets. The transport and delivery of miRNAs by using exosomes is getting higher attention in parasitology and immunology fields due their capacity to modulate the host immune response. Although, the presence of miRNAs in parasitic exosomes has been largely investigated in helminth infections, it is a promising strategy in protozoan parasites (Nawaz et al., Reference Nawaz, Malik, Hameed and Zhou2019). The design of specific inhibitors against those key miRNAs involved in protozoan parasites infection will facilitate the control of leishmaniasis and other infection diseases. In conclusion, the biological information related to miRNAs, parasite infection and the interplay with the host cells and immune response will illuminate future biomedical research. Since miRNAs have a great potential to lead a new class of theranostic tools, the identification of more specific miRNAs with highly specialized functions might provide novel guidelines for the management of parasitic diseases (Paul et al., Reference Paul, Ruiz-Manriquez, Serrano-Cano, Estrada-Meza, Solorio-Diaz and Srivastava2020).
Acknowledgements
PN gratefully acknowledges the support provided by Fundación La Caixa (LCF/PR/PR13/11080005) and Fundación Caja Navarra, Gobierno Navarra Salud (12/2017), Fundación Roviralta, Ubesol, Government of Navarre, Laser Ebro and Inversores Garcilaso de la Vega S.L and COST Actions CA18217 (ENOVAT) and CA18218. EACS thanks to the Consejo Nacional de Investigaciones Científicas y Técnicas (CONICET) and the Agencia Nacional de Promoción Científica y Tecnológica (ANPCyT), Argentina, [PICT 2016-4649 (PRH 2014-0014) and PICT 2018 03070].
Financial support
This research did not receive any specific grant from funding agencies in the public, commercial, or not-for-profit sectors.
Conflict of interest
None.
Ethical standards
Not applicable.