Introduction
Advanced glycation endproducts (AGE) are products of the Maillard reaction that is known as a typical non-enzymic browning reaction between the free carbonyl group of a reducing sugar and the amine group of a protein(Reference Ames and Caballero1). Louis Camille, a French chemist, first described the Maillard reaction in 1912 and showed the effects of AGE that occurred during heat processing and storage of foods on food quality. Although the Maillard reaction in foods has been studying by the food industry for more than a century, the products of the Maillard reaction produced by a similar glycation process in the human body were recognised only about 50 years ago. The description of a high concentration of glycosylated Hb in diabetic patients showed that AGE might have detrimental effects in humans(Reference Rahbar, Blumenfeld and Ranney2). Today, the study of AGE including their sources and effects on health has been a promising research area(Reference Poulsen, Hedegaard and Andersen3).
AGE are a group of complex and heterogeneous molecules, sharing some common features such as covalent cross-link formation among proteins, the effect of transforming the colour of some food products into yellow-brown colours (‘browning’ effect) and fluorescence formation(Reference Singh, Barden and Mori4). Based on their characteristics, AGE are classified into two groups: AGE with fluorescence and cross-links including pentosidine, crossline, 2-(2-furoyl)-4(5)-(2-furanyl)-IH-imidazole, glyoxal–lysine dimer and methylglyoxallysine dimer (MOLD); and AGE without fluorescence (non-fluorescence) and cross-links including N ϵ-(carboxymethyl)lysine (CML), N ϵ-(carboxyethyl)lysine (CEL) and pyrralline(Reference Wu, Huang and Lin5). Although especially food-derived AGE show a great variety of compounds with different molecular weight and chemical structure due to a large variation of precursors in foods, only a few of them have been clearly defined and their amounts in the foods measured(Reference Poulsen, Hedegaard and Andersen3 , Reference Zhang, Huang and Xiao6).
The formation of AGE is based on the Maillard reaction which has generally a three-stage glycation process including initial, intermediate and final(Reference Nursten and Roginski7). The glycation starts when the reducing sugar is covalently bound to an amine group of protein(Reference Sell8). Lysine, arginine and sulfur-containing amino acids are particularly vulnerable to glycoxidation while glucose, fructose, galactose, mannose, ribose and reactive triose intermediates are involved in the endogenous formation of AGE(Reference Ottum and Mistry9). The condensation of a carbonyl group of sugar with an amine group of protein forms an unsteady Schiff base adduct or glycosylamines in hours. Then, the Schiff base transforms into an Amadori product, a steadier and more colourless ketoamine in days. In the intermediate stage, the Amadori product degrades into colourless or yellow advanced products which are highly unsaturated and prone to polymerisation(Reference Sell8). The reactions that form both a Schiff base at the initial stage and Amadori products at the intermediate stage are reversible; however, the reactions at the final stage are irreversible(Reference Snelson and Coughlan10). At the final stage, the Maillard reaction proceeds with dehydration, degradation, oxidation, reduction, condensation and polymerisation of compounds, and Amadori products are converted into highly reactive carbonyl species including dicarbonyls and oxoaldehydes. Therefore, AGE occur over a period of weeks or months (Fig. 1). The accumulation of reactive dicarbonyls, oxoaldehydes or products of glycoxidation or lipoxidation leads to carbonyl stress(Reference Sharma, Kaur and Thind11 , Reference Hodge12). This reaction series depends on different parameters including temperature, pH, humidity and the presence of amine and free carbonyl groups. All these conditions are also responsible for a range of colour, odour, flavour and palatability depending on the formed compound with different chemical structures and biological actions(Reference ALjahdali and Carbonero13).
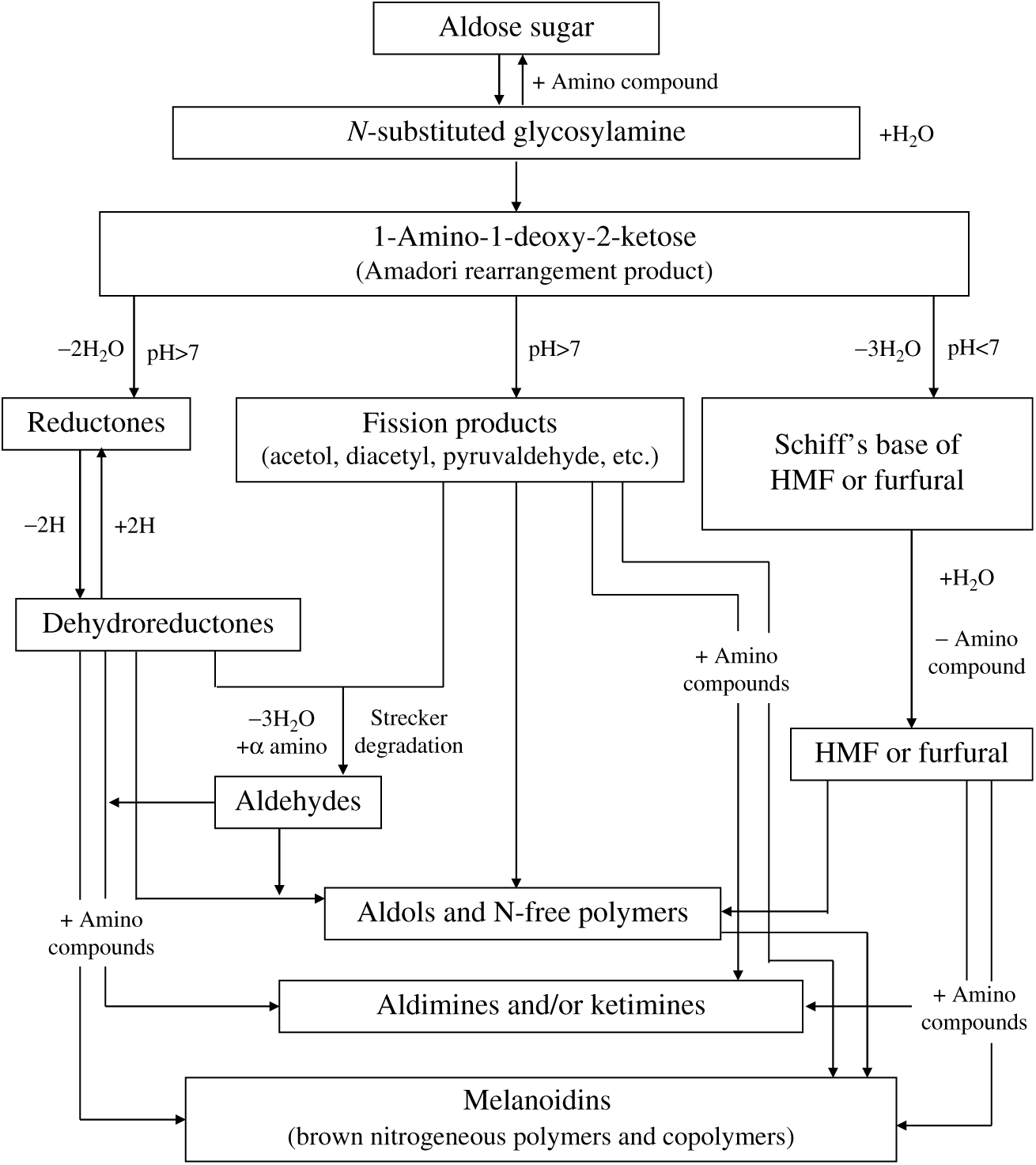
Fig. 1. Pathway of advanced glycation endproduct formation from the Maillard reaction adapted from Hodge (1953)(Reference Hodge12). HMF, hydroxymethylfurfural.
AGE are formed endogenously from the non-enzymic glycation of proteins, lipids and nucleic acids in all body tissues and fluids under physiological conditions, but are also provided from exogenous sources including the diet and tobacco smoke. The body AGE pool reflects both endogenous production and exogenous intake(Reference Palimeri, Palioura and Diamanti-Kandarakis14 ,Reference Ahmed, Luthen and Haussinger15). Recently, the contribution of dietary AGE to the AGE pool of the body has been pointed out when it was recognised that dietary AGE are absorbed and their exposure has increased proportionally(Reference Cordain, Eaton and Sebastian16). It is known that approximately 10 % of dietary AGE are absorbed through the intestine. While one-third of the absorbed AGE are excreted in the urine within 48 h, the other two-thirds remain in the tissues(Reference Koschinsky, He and Mitsuhashi17). AGE with high molecular weight are absorbed more slowly and less efficiently than the AGE with low molecular weight. The degradation of high-molecular-weight AGE by gut proteases is essential for their bioavailability(Reference Koschinsky, He and Mitsuhashi17 – Reference Foerster, Kuhne and Henle19). The rest of the ingested AGE could be found in the colon where they could possibly have an interaction with the bacterial microbiota(Reference Kellow, Coughlan and Savige20,Reference Yacoub, Nugent and Cai21). It has been hypothesised that the Western diet, rich in processed and/or heat-treated foods, fat and sugar, might increase the exposure to AGE and their accumulation in the body due to overnutrition(Reference Cordain, Eaton and Sebastian16).
Although the formation of AGE is a part of normal metabolism, excessively high levels of AGE in tissues and the circulation are described as pathogenic. This is because AGE promote oxidative stress and inflammation by binding with cell surface receptors or cross-linking with body proteins, altering their structure and function(Reference Sharma, Kaur and Thind11 , Reference Hodge12,Reference Tessier and Birlouez-Aragon22 –Reference Birlouez-Aragon, Saavedra and Tessier27). It has been widely accepted that AGE in vivo are related to many health problems including diabetic complications, renal failure, hepatic impairment, CVD, female reproductive dysfunction, neuro-degenerative and eye disorders and even cancer. Therefore, the inhibition of AGE formation and limitation of dietary AGE intake have been suggested to prevent the detrimental effects of AGE on health(Reference Palimeri, Palioura and Diamanti-Kandarakis14 ,Reference Gómez-Ojeda, Jaramillo-Ortíz and Wrobel28–Reference Jiao, Stolzenberg-Solomon and Zimmerman30).
AGE inhibitors are classified into two groups: as synthetic compounds and natural products(Reference Peng, Ma, Chen and Wang31). In general, AGE inhibitors show their inhibitory mechanism by blocking sugar attachment to proteins, attenuating glycoxidation and oxidative stress through trapping or scavenging some intermediates including reactive dicarbonyls, free radicals and N species produced in the process of glycation, delaying and preventing peroxidation of membrane lipids and LDL-cholesterol, reducing the AGE-induced oxidative stress and breaking down formed AGE crosslinks(Reference Jahan and Choudhary32). Synthetic AGE inhibitors consist of aminoguanidine, dimethylbisguanidine (also known as metformin), desferrioxamine, clioquinol, 2-isopropylidenehydrazono-4-oxo-thiazolidin5-ylacetanilide (OPB-9195), N-phenylacyl-1,3-thiazolium bromide (PTB), N-phenylacyl-4,5-dimethyl-1,3-thiazolium chloride, d-penicilline, ibuprofen, indomethacin and acetylsalicylic acid(Reference Brownlee, Vlassara and Kooney33 – Reference Rahbar and Figarola35). Natural AGE inhibitors are vitamins such as vitamin E and lipoic acid, and also phytochemicals such as diferuloylmethane (curcumin), flavanoids and terpenes(Reference Jain and Palmer36 – Reference Sajithlal, Chithra and Chandrakasan39). The investigation of new AGE inhibitors might provide a potential therapeutic approach for the prevention of mainly diabetic complications(Reference Peng, Ma, Chen and Wang31).
In terms of limitation of dietary AGE intake, studies have primarily focused on the determination of AGE contents of foods. A variety of different analytical methods has been used to determine the amount of AGE in foods. The analytical methods are divided into two groups: as instrumental methods including HPLC with diode array detector (HPLC-DAD)(Reference Rufián-Henares, Guerra-Hernández and García-Villanova40), HPLC with fluorescence detector(Reference Hartkopf, Pahlke and Lüdemann41 – Reference Ahmed, Argirov and Minhas44), HPLC with UV detection (HPLC-UV)(Reference Wellner, Huettl and Henle45), liquid chromatography−tandem MS (LC-MS/MS)(Reference Ahmed, Mirshekar-Syahkal and Kennish46 – Reference Hegele, Parisod and Richoz48), ultra-performance liquid chromatography−tandem MS (UPLC-MS/MS)(Reference Scheijen, Clevers and Engelen49) and GC-MS(Reference Charissou, Ait-Ameur and Birlouez-Aragon50 ,Reference Petrovic, Futas and Chandoga51); and also as immunochemical methods including ELISA based on antibodies for different AGE(Reference Uribarri, Woodruff and Goodman52 ,Reference Goldberg, Cai and Peppa53). There are some interests and limits of the determination methods of dietary AGE. For instance, determination of AGE by the ELISA method gives only semi-quantitative results, and, therefore, it is essential to be aware of the cross-reactivity of the antibodies employed(Reference Charissou, Ait-Ameur and Birlouez-Aragon50). In addition to the characterisation of the antibody, the ELISA method should be validated for each matrix(Reference Poulsen, Andersen and Hedegaard18). If this validation step were to be missed, the results of the ELISA would be partially misleading. In the HPLC-DAD method, UV active compounds are needed(Reference Foerster, Kuhne and Henle19). GC-MS, HPLC-UV or LC-MC studies report the results as either only the representative molecule of AGE, particularly CML(Reference Assar, Moloney and Lima47), or the AGE content of just one food product/category(Reference Zhang, Huang and Xiao6 ,Reference Ahmed, Mirshekar-Syahkal and Kennish46). On the contrary, UPLC-MS/MS has the ability to analyse more than one AGE in one run(Reference Scheijen, Clevers and Engelen49). In addition, the differences in reporting the results in terms of units make comparison difficult. For example, while ELISA results are expressed as AGE kilounits/100 g food(Reference Uribarri, Woodruff and Goodman52), LC-MS/MS results are expressed as concentrations(Reference Petrovic, Futas and Chandoga51), such as mg/kg protein or mg/kg food(Reference Poulsen, Andersen and Hedegaard18).
Animal-derived foods are natural dietary sources of AGE even if they are uncooked. The food composition, particularly fat and protein contents, plays an important role in AGE formation. Foods that contain high amounts of fat and protein are generally rich in AGE, and also prone to the formation of new AGE during cooking, compared with foods with high carbohydrate content including vegetables, fruits, whole grains and legumes(Reference Uribarri, Woodruff and Goodman52). The high contents of water, antioxidant vitamins and phytochemicals prevent the formation of AGE, partially in foods rich in carbohydrates(Reference Sharma, Kaur and Thind11).
In addition to the composition of foods, cooking or thermal processing is mainly responsible for the formation of new AGE within foods. Cooking, described as the transfer of energy from a heat source to food, is used for reasons of safety and convenience as well as to enhance sensory characteristics of foods including flavour, texture, colour and appearance since fire was discovered. Different home cooking methods reflect the ways in which the temperature of food is increased(Reference Rosenthal, Caballero, Finglas and Toldra54). Dry and wet cooking or frying procedures are the most commonly used thermal processing techniques and cooking methods(Reference Rosenthal, Caballero, Finglas and Toldra54,Reference Vintilă, Prakash, Martín-Belloso, Keener, Astley, Braun, McMahon and Lelieveld55). In dry cooking including baking, roasting, grilling (broiling) and microwave cooking, the food is directly exposed to heat without a thermal transfer medium such as water or oil(Reference Rosenthal, Caballero, Finglas and Toldra54). In microwave cooking, energy is transferred by electromagnetic radiation with a typical wavelength of 300 mm(Reference Rosenthal, Caballero, Finglas and Toldra54). In contrast to dry cooking, a liquid transfer medium including water and milk is used in order to thermal transfer in wet cooking methods such as boiling, poaching, stewing, braising and steaming. In frying (such as shallow frying and deep frying), oil or fat is used instead of water(Reference Vintilă, Prakash, Martín-Belloso, Keener, Astley, Braun, McMahon and Lelieveld55). Despite the desirable effects of cooking in terms of food safety and enhancement of sensory characteristics and nutritional value, cooking might also lead to the occurrence of AGE(Reference Uribarri, Woodruff and Goodman52 , Reference Nayak, Liu and Tang56 – Reference Zhao, Liu and Lai59). The parameters of cooking that affect the formation of AGE are listed as the cooking temperature, cooking period, the degree of humidity, pH and presence of amine and free carbonyl groups, metals and also antioxidants (Fig. 2)(Reference Rufián-Henares, Guerra-Hernández and García-Villanova40 ,Reference Goldberg, Cai and Peppa53, Reference Nowotny, Schröter and Schreiner60). The present article aimed to review the literature about the effects of different cooking methods and conditions on the AGE content of food and AGE formation mechanisms using a comprehensive approach.

Fig. 2. Factors that affect the formation of advanced glycation endproducts. For a colour figure, see the online version of the paper.
Methods
The experimental research that determined the AGE content of foods occurring during different cooking conditions was selected to design the theme of this review. MEDLINE (http://www.ncbi.nlm.nih.gov/pubmed) that offers access to more than 26 million articles was used to specify the studies. Only research articles in the English language and that were conducted between 2004 and 2019 (last 15 years) were included in the study. The following keywords were used: ‘advanced glycation endproducts and cooking’, ‘advanced glycation end products content of foods’, ‘formation of advanced glycation endproducts in meat’, ‘determination of advanced glycation endproducts by LC-MS/MS in food’, ‘determination of advanced glycation endproducts by ELISA in food’, ‘determination of advanced glycation endproducts by HPLC in food’ and ‘determination of nepsilon (carboxymethyl) l lysine in food’. A total of 397 different articles were obtained with the given keywords, and twenty-three of them that met the criteria were selected. Exclusion was based on being a review article or not including any cooking methods. Research selection was done with the agreement of all reviewers. A schematic representation of the selection process of studies is given in Fig. 3.

Fig. 3. Schematic representation of study selection process. LC, liquid chromatography.
Results
A total of twenty-three studies were included in this review as a result of the literature search. Studies that determined the AGE content of various foods (n 5), meat and meat products (n 6), nuts (n 1), pastry products (n 7) and bread (n 4) were included in the present review. From all the studies, five studies gave the results as change in inhibition of AGE. Therefore, based on different cooking methods and conditions, the AGE contents and the methods of detecting the amount of AGE for certain foods in eighteen studies are given in Table 1. Studies that were included in this review used the following methods to analyse the AGE content of food products: HPLC (four studies), ultra-performance liquid chromatography–tandem MS (UPLC-MS/MS) (six studies), LC-MS/MS (seven studies), ELISA (five studies) and a combination of ELISA and LC-MS/MS (one study).
Table 1. Advanced glycation endproduct (AGE) contents of certain foods, based on different cooking methods and conditions

UPLC-MS/MS, ultra-performance liquid chromatography tandem MS; CML, N ϵ-(carboxymethyl)lysine; CEL, N ϵ-(carboxyethyl)lysine; LC-MS/MS, liquid chromatography−tandem; UPLC-MS, ultra-performance liquid chromatography MS; ND, not detected; MG-H1, methylglyoxal-derived hydroimidazolone.
Advanced glycation endproduct content of meat and meat substitutes
Meat, poultry and fish are the food groups for which the AGE contents have been mostly analysed. The effects of different cooking methods used for meat and meat substitutes on AGE formation have also been examined by different research groups. Scheijen et al.(Reference Scheijen, Clevers and Engelen49) found that sausages made of ground meat had high AGE contents as a result of frying of sausages. In the frying process, the heat firstly affects the frying oil; the food uses the heat of hot frying oil; and consequently, the interaction between hot frying oil and food is responsible for the formation of AGE(Reference Chao, Hsu and Yin61). In the case of ground meat, the effect of grinding may increase lipid and protein oxidation, the products of which may consequently turn into carbonyl intermediates, as a result of higher exposure to air which plays a role in AGE formation together with the heat(Reference Kanner62). In addition, Assar et al.(Reference Assar, Moloney and Lima47) portioned the samples of ground beef as 50 g and fried in a pan, previously heated under medium temperature for 2 min following high temperature for 1 min, or boiled them in a boiling 250 ml of water for 3 min. At the end of the study, the CML amount in fried meats was found to be two times higher than the CML amount of boiled meats (11·2 and 5·02 mg/kg food, respectively). The reason for the high CML content of fried samples originates from more lipid oxidation due to the higher thermal effect during the frying process.
In a study conducted by Trevisan et al.(Reference Trevisan, de Almeida Lima and Sampaio63), hamburgers that were made of ground beef were cooked with four different cooking methods, such as grilling, boiling, breading/frying (powdered eggs, wheat flour) and baking, for detecting the CML contents. Accordingly, CML content was detected as 0·8 mg/100 g only in meats baked at 300°C whereas it was not detectable following other cooking methods. The low amount of reducing sugar, low fat content (1·6 %) and low temperature (<100°C) in the geometric centre explains undetected CML and the detection of low CML levels in domestic applications(Reference Hull, Woodside and Ames64). In the study of Sun et al.(Reference Sun, Tang and Wang65) on ground beef, they exposed samples to a water-bath or boiled them in water at seven different cooking temperatures (65, 70, 75, 80, 85, 90 and 100°C). This study showed that the CML amount in ground beef increased steadily due to the increased temperature. Furthermore, the samples cooked under high temperatures contained more CML when the same cooking periods under different temperatures were applied. For example, cooking at 65°C for 60 min caused the increase of CML content by 50 %; cooking at 75°C increased by 154 %, cooking at 85°C increased by 263 % and cooking at 100°C increased by 361 % with the same cooking period. This study also showed that the CEL levels increased with cooking period and temperature. Moreover, it was shown that the CEL and CML contents of raw and heat-applied ground beefs had a strong linear relationship (r 2 0·920), which proved that the contents of both CEL and CML were affected by heat application in the same way and these two compounds could occur via similar metabolic pathways consequently(Reference Sun, Tang and Wang65).
In a study aimed to determine the AGE contents of different meat types using a HPLC method, Chen & Smith(Reference Chen and Smith66) cooked meats with frying, broiling and baking methods until the internal temperature of meats reached a certain temperature. These degrees were determined as 71°C for beef and pork, as 74°C for chicken, and as 63°C for salmon and tilapia. The samples were taken from the inner and outer parts of the meats and the AGE contents of the meats were analysed. Except for tilapia, there was no significant difference in the inner parts of the fried meats (P> 0·05). However, the AGE contents of the outer parts of the meats showed differences according to the uncooked amounts of all meats (P< 0·05). The difference probably originates from the fact that the water-soluble precursors are transferred to the outer parts of the meats during the cooking period and form the AGE(Reference Chen and Smith66). The CML contents of beef, pork and chicken meats were found higher in broiling and frying compared with in baking. The explanation of this situation is that the cooking temperature required for the desired internal temperatures of the meats was higher in the broiling and frying methods than the baking method. As the foods are indirectly exposed to hot air in the baking method but they are directly exposed to the heat source in the frying method, it can cause a decreasing CML content in baked meats, rather than fried meats(Reference Chen and Smith66). Mitra et al.(Reference Mitra, Lametsch and Greco67) determined how thermal treatments of pork, with different combinations of time and temperature (included raw samples (control), 58, 80, 98 and 160°C for 72 min, 118°C for 8 min and 58°C for 17 min) affect the AGE levels, and found that CML showed a negligible amount in control, but increased with cooking temperature.
In the study of Uribarri et al.(Reference Uribarri, Woodruff and Goodman52), the CML content of 549 foods was detected by using the ELISA method, and it was shown that the AGE contents of the foods changed under different water and temperature conditions. For instance, it was observed that scrambled eggs, cooked in an open pan using a medium-low temperature with olive oil for 2 min, contained levels of AGE by half, when compared with eggs cooked with the same method but using a high temperature with olive oil for 1 min (97 and 243 kU/100 g, respectively). In parallel with this result, the same results were found for scrambled eggs cooked using medium-low temperatures with margarine for 2 min and cooked using high temperatures with margarine for 1 min (63 and 123 kU/100 g, respectively). Chicken breasts, poached using medium temperatures for 7 min (1101 kU/100 g) or steamed using medium temperatures in foil for 15 min (1058 kU/100 g), include one-quarter less AGE than the chicken breasts roasted for 45 min (6639 kU/100 g). In all food categories, the foods exposed to the applications of high temperatures and low moisture contained more AGE than the foods prepared under low temperatures or high moisture. For this reason, more formation of AGE was observed in the cooking methods of frying, broiling, grilling and roasting, when compared with the cooking methods of boiling, poaching, stewing and steaming. As the cooking period is relatively shorter for cooking with a microwave (6 min or shorter), the AGE content did not increase by the same level, as occurred with other dry heat cooking methods. In the study, while the CML amounts of beef steak were found as 7479 and 7416 kU/100 g, respectively, by cooking with broiling and grilling, the CML content of beef steak was found to be 2687 kU/100 g after cooking in a microwave oven for 6 min. Likewise, the CML content of salmon fillets was determined as 3347 kU/100 g by cooking with the method of broiling and as 912 kU/100 g by cooking with the method of a microwave(Reference Uribarri, Woodruff and Goodman52).
Goldberg et al.(Reference Goldberg, Cai and Peppa53) determined the AGE content of 250 foods by using the ELISA method. For this purpose, they categorised foods into three groups as fat, protein and carbohydrate based on their macronutrient contents, and then CML-like AGE contents of foods were analysed. It was shown that the foods in the fat group had the highest AGE content with a mean of 100 (se 19) kU/g, and the protein group including meat and meat substitutes had AGE content with a mean of 43 (se 7) kU/g. It was reported that the carbohydrate group had the lowest AGE content, with a mean of 3·4 (se 1·8) kU/g. Based on these results, it can be said that there is a relationship between macronutrient composition and the AGE content of a food. The reason why the fat and protein groups have higher AGE contents could be explained by the release of free radicals in high levels as a result of various lipo-oxidation reactions during the cooking process. The AGE contents detected in all food categories were associated with cooking temperature, cooking period and the presence of moisture. Broiling (at 225°C) and frying (at 177°C) caused the highest levels of AGE formation, followed by roasting (at 177°C) and boiling (at 100°C). According to the data obtained from the study, it was reported that the temperature and cooking method are more efficient than the cooking period in the formation of AGE. For example, the AGE contents of the meats broiled or grilled at 230°C for a short time had a higher AGE content compared with meats boiled in a liquid state for a longer time. It was also shown that the AGE content of broiled beef for 15 min was three times higher than the AGE content of boiled beef for 1 h (60 and 22 kU/g, respectively). Likewise, the AGE content of broiled chicken breast meat for 15 min was obtained as 5250 kU AGE while the AGE content of boiled chicken breast meat for 1 h was 1000 kU AGE(Reference Goldberg, Cai and Peppa53).
Roldan et al.(Reference Roldan, Loebner and Degen68) added flavour-enhanced solution (d-glucose, d-ribose, l-cysteine and thiamine) into lamb loins and cooked the meats in a vacuum pack at 60°C for 12 h with the sous-vide method or cooked the meats in the oven using dry air at 180°C until the internal temperature reached 73°C. The CML amounts of the meats were found relatively low and did not vary due to the flavour-enhanced solution. In the study of Scheijen et al.(Reference Scheijen, Clevers and Engelen49) the cooking of beef using the stewing method with wine-vinegar sauce resulted in a higher AGE content. This confirms that reducing sugars have an important role in the formation of AGE. The heat causes more amino acids to be released from food, the reducing sugar reacts with the released amino acids, and thereby resulted in the formation of AGE(Reference Nowotny, Schröter and Schreiner60).
Advanced glycation endproduct content of breads
Assar et al.(Reference Assar, Moloney and Lima47) determined the CML contents of crusts and crumbs of baked white and whole-wheat breads. Accordingly, the CML contents of the crusts of both bread types are higher than the CML contents of the crumbs (for white bread, 37·1 and 2·58 mg/kg, respectively; for whole-wheat bread, 46·1 and 4·45 mg/kg, respectively)(Reference Assar, Moloney and Lima47). While baking the breads, the surface temperature of dough can reach 230–250°C, which causes the formation of the crust of the bread and the evaporation of water on the surface. Thereby, the combination of decreased moisture and high temperature facilitates the formation of the Maillard reaction on the surface. On the other hand, the internal temperature of the bread is nearly at 100°C and the moisture is higher than the surface(Reference Wirtz and Cauvain69). This causes the crumb of the bread to have ten times lower CML levels, when compared with the crust of the bread(Reference Van de Merbel, Mentink and Hendriks43). Zhou et al.(Reference Zhou, Lin and Jin70) also determined the CML and CEL contents of different kind of breads including white, roughage and wholemeal with the UPLC–MS/MS method, and found CML contents to be 16·2 (sd 0·9), 27·4 (sd 1·7) and 17·2 (sd 0·7) mg/kg, respectively, and CEL contents to be 4·69 (sd 0·19), 6·01 (sd 0·16) and 5·20 (sd 0·28) mg/kg, respectively.
In another study by Mildner-Szkudlarz et al.(Reference Mildner-Szkudlarz, Siger and Szwengiel71), breads that contained phenolic compounds such as catechin, quercetin, gallic, ferulic and caffeic acids were baked at 180°C for 20 min with the aim of determining antiglycation activity of phenolic compounds, and it was shown that phenolic compounds significantly reduced CML content (31·77–87·56 %) even at the lowest concentrations, excluding ferulic acid. The highest reduction in CML levels was obtained in breads that contained catechin due to its structure–antioxidant activity relationship, its thermal stability, and its reactivity with ϵ-lysine side chains. The study also compared the bread crust and bread crumb in terms of CML levels and showed that bread crust contained 49·71 mg/kg CML and bread crumb contained 15·09 mg/kg CML. The three times greater value of CML in the bread crust over the crumb was explained with the lower moisture content of the dough surface and the higher processing temperature, favouring the Maillard reaction. Likewise, Teng et al.(Reference Teng, Li and Yu72) added naringenin, a common flavanone, into the dough of bread and assessed its impact on the formation of AGE in bread crust. They showed that an increase in naringenin content of bread (0·25–1 %, w/w) inhibited the formation of CML and total fluorescent AGE significantly (9·67–54·27 and 11·79–35·19 %, respectively). In accordance with this study, Lin et al.(Reference Lin, Tan and Leong73) also found that the incorporation of quercetin in steamed bread inhibited fluorescent AGE formation in breads (0·05–0·2 %).
Advanced glycation endproduct content of pastry products
Treibmann et al.(Reference Treibmann, Hellwig and Hellwig74) determined the AGE (CML, CEL and methylglyoxal-derived hydroimidazolone (MG-H1) as important lysine and arginine AGE) content of cookies that were baked in a laboratory drying oven for 10 min at 175°C. The cookies that contained fructose, glucose, honey and banana had similar CML contents (6·22 (sd 0·71), 7·9 (sd 1·5), 6·940 (sd 0·057) and 7·67 (sd 0·18) mg/kg cookie, respectively). These results showed that there was not significant difference between glucose and fructose regarding the formation of CML during the baking process. This might be explained by the similar degradation to CML both in fructosyllysine and glucosyllysine(Reference Hinton and Ames75). In contrast to the CML content of cookies, the cookies with the highest fructose content had the highest MG-H1 and CEL concentrations. MG-H1 and CEL formation from fructose could be an indicator of the ‘fructose pathway’ of the Maillard reaction. In the study of Cheng et al.(Reference Cheng, Jin and Zhang76), cookies baked using various temperature–time regimens (155°C for 6, 11, 16, 21 and 31 min; 180°C for 4, 6, 11, 16 and 21 min; 205°C for 4, 6, 8, 11 and 16 min; 230°C for 1·5, 3, 6, 8 and 11 min) in a commercial convection oven were analysed in terms of CML content. The highest level of CML was found in the cookies baked at 230°C for 1·5 min (118·05 (sd 0·21) mg/kg). It was also shown that CML was prone to form at a relatively high temperature range (205 to 230°C).
Navarro & Morales(Reference Navarro and Morales77) baked biscuits with added hydroxytyrosol, olive leaf extract, quercetin and gallic acid at different temperatures (180 and 200°C) for different times (20 and 10 min) in order to investigate antiglycative activity. They did not find significant differences on the formation of CML that is formed by the reaction between glyoxal and the ϵ-amino group of lysine among the biscuit samples with different phenol compounds. The CML formation precursor, glyoxal, has different ways of formation including the oxidation of fructosyl-lysine, the oxidation of glucose or lipid peroxidation. In this biscuit model, the contribution of lipid oxidation to CML formation cannot be eliminated. Hence, maybe there was another source of reactants for the CML formation which cannot efficiently be delayed by the phenol compounds added in the formulation. It was also found that the amount of CEL formed by the reaction between methylglyoxal and lysine was higher than CML due to the abundance of dicarbonyl methylglyoxal in the biscuit models. In another biscuit model, Liu et al.(Reference Liu, Chen and Zhang78) assessed the inhibitory effects of highland barley bran extract, which contains several phenolic acids, such as caffeic, syringic, p-coumaric, ferulic and sinapic acids, on AGE levels formed during cooking, and they demonstrated that highland barley bran extract can potentially control CML formation during food processing, which was largely attributed to its antioxidant activities and radical-scavenging capacities. Mildner-Szkudlarz et al.(Reference Mildner-Szkudlarz, Siger and Szwengiel79) aimed to determine the effect of different ingredients including salt, baking powder and protein-rich components and grape by-products to muffins that were baked at 180°C for 20 min on CML content by using the HPLC method. They showed that ingredients reduced the CML content of muffins from 50 to 86 %. The highest reduction in CML was observed with the use of all ingredients simultaneously as a consequence of the synergistic effects in the muffin formula. Because of the metal ion-mediated degradation of fructose-lysine, higher amounts of CML was detected in muffins that included raw cane sugar as an ingredient than refined sucrose. With the appropriate levels of grape by-products, CML levels were decreased in muffins. It was reported that phenolic compounds could decrease the CML content of foods in different situations(Reference Chen, Madl and Smith80). In a study of Teng et al.(Reference Teng, Liu and Hu81), it was demonstrated that cookies (baked at 180°C for 20 min) fortified with naringenin, naringin, hesperetin and dihydromyricetin had lower levels of CML and fluorescent AGE.
Srey et al.(Reference Srey, Hull and Connolly82) analysed the CML and CEL contents in different cake models, and showed that the cake with glucose produced the higher CML level than the cake with fructose. The explanation could be that CML formation from the Amadori rearrangement products may be more important than CML formation from the Heyns rearrangement products. Another potential explanation is that the oxidation of glucose generated more glyoxal than the oxidation of fructose. It was also found that CEL was highest in the cakes baked using fructose, followed by glucose, refined sucrose, and unrefined sucrose as the fructose oxidation resulted in a greater yield of methylglyoxal, known as a reactive carbonyl compound, than glucose. They also compared sugar and lipid precursors, and found a 3-fold lower level of CML in the model cake prepared using the low level of oil (molar ratio lysine/oil = 1:1) compared with the high level of oil (molar ratio lysine/oil = 1:50). The cake including refined sucrose generated a 1·3-fold higher level of CML than the cake that did not include refined sucrose. This proved that sucrose affected CML formation to a lesser degree than oil, probably because of the generation of glyoxal via lipid oxidation being greater than that produced via sucrose oxidation. Srey et al.(Reference Srey, Hull and Connolly82) also investigated the effects of AGE inhibitors and showed that ferulic acid, thiamine and thiamine derivatives inhibited the formation of CML and CEL. This may be attributed to the inhibition of the intermediate product including glyoxal and methylglyoxal. Powerful antioxidants including tocopherol and rutin (rutoside) did not show any effects on AGE formation because of the insolubility in the buffer system, as well as thermal baking instability.
Advanced glycation endproduct content of grains and legumes
A study that assessed the AGE contents of frequently consumed foods in Japan showed that the average glucose-driven AGE amount of fried noodles was 25 510 U/meal(Reference Takeuchi, Takino and Furuno83). This study also demonstrated that pasta cooked for 8 min had 112 CML kU/100 g whereas pasta cooked for 12 min had a higher content of AGE (242 CML kU/100 g). On the contrary, rice cooked for 10 min had relatively low levels of AGE (9 CML kU/100 g) compared with cooked pasta(Reference Uribarri, Woodruff and Goodman52). In line with this study, Scheijen et al.(Reference Scheijen, Clevers and Engelen49) also showed that boiled rice had 0·07 mg CML/100 g while boiled and then fried rice had 0·96 mg CML/100 g. In the case of legumes, it was shown that red kidney beans cooked for 1 h had 298 CML kU/100 g which was almost three times higher than the AGE content of raw kidney beans (116 CML kU/100 g)(Reference Uribarri, Woodruff and Goodman52).
Advanced glycation endproduct content of fruits and vegetables
There is limited evidence about the AGE content of fruits and vegetables in the literature. Uribarri et al.(Reference Uribarri, Woodruff and Goodman52) showed that the AGE content of baked banana was 45 kU CML/100 g. They also showed that AGE contents of various grilled vegetables including eggplant, broccoli, carrots and pepper ranged between 226 and 261 CML kU/100 g.
Advanced glycation endproduct content of nuts
Zhang et al.(Reference Zhang, Huang and Xiao6) roasted almonds under six different temperature degrees (129, 138, 146, 154, 168 and 182°C) for different periods, and analysed the amounts of free AGE and free plus bound AGE in almonds using LC-MS/MS. While CML and CEL were detected in raw almonds, no pyrralline was detected. However, pyrralline was detected in all roasted almonds. CML and CEL can occur with lipid oxidation, besides the Maillard reaction(Reference Fu, Requena and Jenkins84). Almonds are typically stored at temperatures <35°C in the harvest period until the moisture of almond seeds reaches 5–8 %. For this reason, CML and CEL amounts of raw almonds, which are rich in fat, can originate from fat oxidation. Pyrralline, the major output of the Maillard reaction, consists of lysine and 3-deoxyglucosulose. The Maillard reaction typically needs a high temperature and low moisture. This situation explains why there is pyrralline only in roasted almond samples. It was observed that roasting of almonds under different temperatures increased the formation of CML, CEL and pyrralline significantly; however, there was no significant correlation between AGE and roasting temperature. After roasting, free CML levels increased by 50 (23·3–73·2) % and free CEL levels increased by 120 (37·7–177·9) %, total CML and CEL levels increased by 186 (91·1–333·6) % and 413 (187·5–1425) %, respectively. In roasted almonds, pyrralline was found in the amount of 64 (30·7–84·4) % as the major AGE(Reference Zhang, Huang and Xiao6). In addition, Uribarri et al.(Reference Uribarri, Woodruff and Goodman52) found the AGE contents of roasted almonds, cashews and chestnuts to be 6650 CML kU/100 g, 9807 AGE kU/100 g and 5353 AGE kU/100 g, respectively.
Conclusion
The formation of AGE in foods is subjected to the factors such as the AGE formation rate and variety, the composition of food, the presence of precursors, transition metals, and pro- and antioxidants. Cooking period, cooking temperature, the concentration of reactants, the presence of water and pH are important factors for determining the Maillard reaction rate(Reference Vlassara and Uribarri85). According to the results of the studies, in which the AGE contents of foods were detected with different cooking methods, instead of roasting, grilling and frying, with appropriate culinary techniques including low temperature and high moisture such as stewing, poaching and boiling, low AGE levels can easily be achieved in the foods. Moreover, marination with lemon or vinegar, that also affects the pH level of the food, can decrease the formation of AGE in foods(Reference Uribarri, del Castillo and de la Maza86). By choosing the appropriate cooking methods, the amount of dietary AGE intake can be decreased significantly. On the other hand, a decrease in the consumption of fat-rich foods including red meat, processed foods and fast foods along with an increase in the consumption of fruits, vegetables, whole grains and legumes, not only decrease AGE exposure but also important nutritional goals can be achieved(Reference Snelson and Coughlan10). The decrease in dietary AGE intake is especially important for groups who have chronic diseases such as diabetes and CVD. In terms of this population group, especially the nutritional and pharmaceutical targets for the prevention of diseases such as the inhibitors of their action in the body and enhancers of their degradation are important elements of AGE prevention.
As Gerrard(Reference Gerrard87) pointed out, there is still much work that remains to be done about AGE, especially the advent of more sensitive methodology in order to monitor the formation and degradation of AGE in food. Although there are many different studies to determine the amount of AGE in foods, there is not yet a common method for determining the AGE content of food products. This leads to differences in the results of studies and the inability to compare these results correctly. Therefore, a common method for determining the amount of AGE in foods should be developed. In addition, AGE contents of foods were determined as different markers including CML, CEL, pentoisidine and total amount of AGE in previous studies. A new analytic quantification of AGE in food will also remove this disparity to ensure a more precise result in detecting AGE levels in foods.
Acknowledgements
The present review received no specific grant from any funding agency, commercial or non-profit sectors.
The authors’ responsibilities were as follows: Z. B. and A. A. contributed to the conception of the literature search strategy. E. I. and Z. B. undertook the literature search and wrote the manuscript. E. I., Z. B. and A. A. critically appraised the document at all stages. All authors were responsible for the final content.
None of the authors has any conflicts of interest.