Gestational diabetes mellitus (GDM), defined as glucose intolerance first recognised during pregnancy, is a major public health issue, with an estimated 18·4 million births affected globally per annum(Reference Cho, Shaw and Karuranga1). In many countries, rates of GDM have increased steadily over recent decades(Reference Lavery, Friedman and Keyes2), a trend that continues unabated in the absence of effective preventative strategies(Reference Plows, Reynolds and Vickers3). GDM not only increases the risk of major obstetric and perinatal complications, such as pre-eclampsia, stillbirth, macrosomia, shoulder dystocia, birth trauma and neonatal encephalopathy(Reference Reece4), but is also associated with long-term health risks for women and their infants. Infants exposed to GDM also have increased incidence of obesity and insulin resistance in childhood, and impaired glucose tolerance and type 2 diabetes mellitus in adulthood; risks that are further increased in those born large for gestational age(Reference Kim, Sharma and Callaghan5–Reference Dabelea, Mayer-Davis and Lamichhane7).
The mechanisms underlying these long-term consequences for infants exposed to GDM are not well understood. Infancy is a critical period of development, characterised by rapid changes in growth, nutrition and feeding patterns, that have an important influence on growth and body composition throughout childhood and beyond(Reference Thompson and Bentley8,Reference Butte, Hopkinson and Wong9) . For example, breast-feeding protects against childhood obesity(Reference Arenz, Ruckerl and Koletzko10), whereas early introduction of formula milk or bottle feeding is associated with more rapid infant weight gain and subsequent increased risk of childhood obesity(Reference Li, Magadia and Fein11,Reference Dennison, Edmunds and Stratton12) . Similarly, early introduction of solids and greater avidity for food at 3 months of age are associated with increased adiposity in later life(Reference Moorcroft, Marshall and McCormick13–Reference Shepard and Chandler-Laney16). Therefore, nutrition, growth and feeding behaviours in infancy appear to have a potential role in mediating the risk of later obesity and metabolic disease.
The association between GDM and offspring obesity and diabetes may be due to effects on growth, nutrition and appetite in infancy, either as a result of altered nutrition in utero or postnatally via breast milk composition. For example, rats exposed to maternal diabetes in pregnancy demonstrate hyperinsulinaemia, and exposure to breast milk of dams with GDM has been shown to alter hypothalamic function, which may influence satiety centres and regulation of body weight and metabolism(Reference Fahrenkrog, Harder and Stolaczyk17,Reference Plagemann, Harder and Janert18) . In humans, GDM has been associated with lower breast-feeding duration, early introduction of cows’ milk(Reference Hummel, Vehik and Uusitalo19,Reference Dugas, Perron and Marc20) and greater weight gain in the first 3 months of life(Reference Logan, Gale and Hyde21,Reference Kramer, Hamilton and Ye22) .
To date, the association between GDM and feeding, nutrition and growth in infancy has not been systematically assessed. This information may be important in guiding clinical care of infants exposed to GDM and in designing intervention trials to reduce their risks of obesity and metabolic disease. Thus, we undertook a systematic review and meta-analysis to evaluate the impact of GDM on infant feeding patterns and behaviour, nutritional intake and growth in the first 2 years after birth.
Methods
This systematic review was conducted following the principles of the Preferred Reporting Items for Systematic Reviews and Meta-Analyses statement. The protocol was registered in PROSPERO (CRD42018115212, http://www.crd.york.ac.uk/PROSPERO/).
Search strategy
We searched MEDLINE, Web of Science, Embase, CINAHL and Cochrane Central Register of Controlled Trials (CENTRAL) database using key words, MeSH terms and Emtree headings including gestational diabetes, infant nutrition, breast-feeding, infant formula, weaning, appetite, feeding behaviour, energy intake, food frequency, body size, BMI, skinfold thickness and body composition. We also searched for alternative terms for the main concepts using phrasing and truncation (online Supplementary File S1). The search was limited to studies involving humans and with abstracts. We did not limit the search to any language or year of publication. We also hand searched references lists in eligible studies, review papers and conference abstracts to identify additional items. One author identified records through database searching and screened titles and abstracts for potential eligibility. Two authors then independently assessed the full text for eligibility. Discrepancies were resolved through discussion or consultation with the third author. We used a reference management software Covidence (https://www.covidence.org) to combine search results from different databases and to remove duplicates.
Inclusion criteria
We included all published studies (case–control, cohort and randomised trials) that reported one or more primary or secondary outcomes up to 2 years of age in infants exposed to GDM compared with those not so exposed. We included studies that reported GDM as diagnosed by oral carbohydrate challenge test (any diagnostic criteria), treated or untreated and maternal self-report. Studies that retrospectively collected data on GDM status from hospital records were also included in the review. We excluded uncontrolled case series, unpublished results, conference abstracts and studies primarily about pre-gestational diabetes. We did not limit studies by health care setting or country.
Outcomes
The primary outcomes were energy intake (kJ) and BMI (kg/m2) z-score or standardised score. Secondary outcomes related to feeding patterns and behaviour were: (a) exclusive or predominant breast-feeding at ≥5 months of age, defined as the proportion of infants fed exclusively or predominantly with breast milk (can include medicines, vitamins, oral re-hydration solution and water-based liquids, in addition to breast milk) from birth to at least 5 months of age(23); (b) introduction of formula milk before hospital discharge; (c) continued breast-feeding at 12 months of age, defined as the proportion of children fed any breast milk at 12–15 months of age(24); (d) duration of breast-feeding(25); (e) no breast milk feeding, defined as proportion of infants who did not receive any breast milk at ≤5 months(26); (f) introduction of solid, semi-solid or soft foods before 5 months of age and (g) appetitive scores to assess the appetite-related feeding behaviours, for example, Baby Eating Behaviour Questionnaire for infants <6 months and Child Eating Behaviour Questionnaire for older infants(Reference Llewellyn, van Jaarsveld and Johnson27,Reference Birch, Fisher and Grimm-Thomas28) . Secondary outcomes related to nutritional intake were: (a) breast milk composition including energy (kJ/100 ml), lactose (g/100 ml), protein (g/100 ml) and fat (g/100 ml); (b) minimum diet diversity, defined as the proportion of children who received foods from ≥5 out of eight food groups during the previous day (breast milk, grains, roots and tubers, legumes and nuts, dairy products, flesh foods, eggs, vitamin-A-rich fruits and vegetables, and other fruits and vegetables)(29); (c) food group frequency; and (d) macronutrient intake, including daily intake of protein (g), carbohydrate (g) and fat (g), and percentage of energy from protein, carbohydrates and fats. Secondary growth outcomes were weight (kg), length (cm), abdominal circumference (cm), head circumference (cm), skinfold thicknesses (mm), fat mass (g) and fat-free mass (g) z-scores or standardised scores.
Risk of bias
Two authors independently assessed the risk of bias of each study using the ROBINS-I tool for non-randomised studies of interventions(Reference Sterne, Hernán and Reeves30), a modified version of the ROBINS-I tool for non-interventional observational studies(Reference Bradford, Thompson and Heazell31), or the Cochrane Collaboration’s risk of bias tool for randomised trials(Reference Higgins, Altman and Gøtzsche32). The following bias domains were assessed: (a) recruitment and selection of participants in the study; (b) confounding; (c) ascertainment of exposures; (d) measurement of outcomes; (e) missing data and (f) reporting of results. Discrepancies between authors were resolved through discussion or by consultation with the third reviewer.
Data extraction and analysis
Two authors independently extracted data from included studies using a pre-specified data form. We extracted year of publication, type of study, country of the study, study population, participant characteristics, definition of gestational diabetes used (including diagnostic criteria), treatment, inclusion and exclusion criteria, adjustment for confounding and outcomes specific for the review. Discrepancies between authors were resolved through discussion or by consultation with the third author.
Meta-analysis was performed separately across three age epochs (1–6, 7–12 and 13–24 months) using Review Manager (REVMAN) version 5.3(33). An inverse variance, fixed-effects method was used, based on adjusted estimates where available. If adjusted estimates of exposure effect were not available, meta-analysis was performed using extracted summary statistics, either proportions or mean differences, as appropriate.
If there was more than one report from a single study, only the latest report within each epoch was used in analysis. Exposure effects are presented as OR or standardised mean difference (SMD) with 95 % CI. For continuous data, if median and interquartile range were reported, we estimated mean values and standard deviation to pool the results in meta-analysis(Reference Wan, Wang and Liu34). Statistical heterogeneity was assessed by the χ 2 test and I 2 statistic values. If meta-analysis was not possible, we provided a narrative synthesis of findings.
We planned subgroup analysis for primary outcomes comparing higher and lower degrees of maternal dysglycaemia. We also planned sensitivity analysis, excluding studies with high risk of bias.
Quality of evidence
We used the GRADE approach to assess the quality of evidence for each outcome(Reference Balshem, Helfand and Schunemann35). Two reviewers independently assessed the quality of evidence based on eight assessment criteria(Reference Ryan and Hill36). Observational studies were initially assigned a low quality of evidence and randomised controlled trials a high quality of evidence. The quality level was downgraded if: (a) one or more studies had uncertain or high risk of bias for several domains; (b) there was evidence of substantial heterogeneity (I 2 statistic value >50 % and low P value for χ 2 test)(Reference Ryan and Hill36); (c) there was indirectness in reporting of participants, exposure, comparison and outcomes or (d) there was imprecision of results due to a total number of events <300 (for dichotomous outcomes), total number of participants <400 (for continuous outcome) and wide confidence intervals. We upgraded the quality by one level if there were only observational studies with no major threats to validity and there was evidence of large exposure effect (for dichotomous outcomes, OR > 2 or <0·5; for continuous data, SMD > 0·8)
Results
Search results
We identified 5445 citations and removed 2121 duplicates. The remaining 3324 citations were screened for title and abstract. Of these, 3135 citations were irrelevant and were excluded. Following full-text screening of 189 citations, 163 were excluded and twenty-six publications (twenty-five studies) were included (Fig. 1). One study published in Chinese(Reference Zhao, Ma and Zhang37) and one published in German(Reference Hummel, Hummel and Knopff38) were translated to English. Two studies reported secondary analysis of data from the Infant Feeding Practices study II(Reference Wallenborn, Perera and Masho39,Reference Weisband, Rausch and Kachoria40) . Four publications (three studies) had no extractable data for any outcomes(Reference Zhao, Ma and Zhang37,Reference Crume, Ogden and Daniels41–Reference Hakanen, Saha and Salo43) ; thus, twenty-two studies comprising 301 622 infants were included in the meta-analysis.

Fig. 1. Study identification, inclusion and exclusion.
Characteristics of the selected studies
All the included studies were observational, with the majority being cohort studies; thirteen were prospective(Reference Hummel, Vehik and Uusitalo19,Reference Zhao, Ma and Zhang37,Reference Vohr and McGarvey44–Reference Uebel, Pusch and Gedrich54) , eleven were retrospective(Reference Dugas, Perron and Marc20,Reference Hummel, Hummel and Knopff38–Reference Hakanen, Saha and Salo43,Reference Liu, Li and Sun55–Reference Finkelstein, Keely and Feig59) and one was a retrospective case–control study(Reference Konig, Junginger and Reusch60) (Table 1). All but three studies(Reference Hummel, Hummel and Knopff38,Reference Vohr and McGarvey44,Reference Krishnaveni, Hill and Leary45) were published after 2010. The studies were conducted in both developed and developing countries, including the USA, Germany, Finland, Sweden, Greece, Portugal, the UK, Canada, Australia, Singapore, Israel, China, India, Vietnam, Brazil, Colombia, Kenya and South Africa, and were carried out in various settings, such as tertiary hospitals, university hospitals, community clinics and research centres.
Table 1. Characteristics of included studies
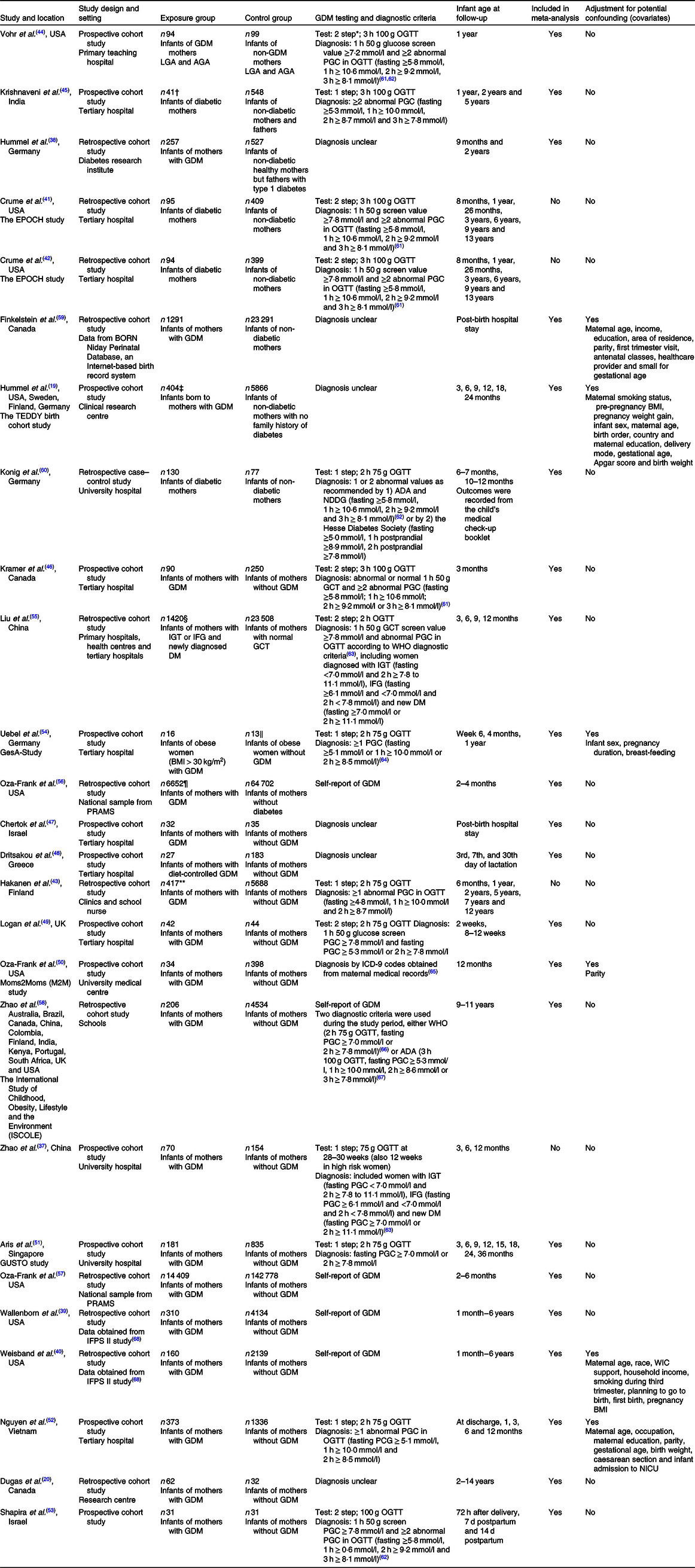
GDM, gestational diabetes mellitus; LGA, large for gestational age; AGA, appropriate for gestational age; OGTT, oral glucose tolerance test; PGC, plasma glucose concentration; EPOCH, Exploring Perinatal Outcomes among Children; BORN, The Better Outcomes Registry and Network; TEDDY, The Environmental Determinants of Diabetes in the Young; ADA, American Diabetic Association; NDDG, National Diabetes Data Group; GCT, glucose challenge test; IGT, impaired glucose tolerance; IFG, impaired fasting glucose; DM, diabetes mellitus; PRAMS, The Pregnancy Risk Assessment Monitoring System; ICD, International Classification of Diseases; GUSTO, Growing Up in Singapore Towards Healthy Outcomes; IFPS, The Infant Feeding Practices Study; WIC, The Women, Infants and Children’s program; NICU, neonatal intensive care unit; T1D, type 1 diabetes.
* In the two-step approach, women are screened at 24 to 28 weeks’ gestation by a non-fasted 1 h, 50 g oral glucose challenge test; women who screen positive for this test subsequently undergo a diagnostic OGTT.
† n 41 Offspring of diabetic fathers.
‡ n 292 Infants born to mother with T1D, n 464 Infants who have mother without T1D but a father and/or sibling with T1D.
§ n 2229 Infants born to mothers with positive GCT and normal OGTT.
‖ n 15 Infants born to lean mothers without GDM.
¶ n 1401 Infants born to mothers with pre-gestational diabetes.
** n 53 Infants born to mother with T1D are included in the respective cohorts but not included for analysis.
GDM was diagnosed using a one-step 2-h 75 g oral glucose tolerance test in six studies(Reference Zhao, Ma and Zhang37,Reference Hakanen, Saha and Salo43,Reference Aris, Soh and Tint51,Reference Nguyen, Binns and Lee52,Reference Uebel, Pusch and Gedrich54,Reference Konig, Junginger and Reusch60) ; one-step 3-h oral glucose tolerance test in one study(Reference Krishnaveni, Hill and Leary45); 50 g polycose screen followed by 2-h 75 g oral glucose tolerance test in two studies(Reference Logan, Emsley and Jeffries49,Reference Liu, Li and Sun55) and 3-h oral glucose tolerance test in four studies(Reference Crume, Ogden and Daniels41,Reference Crume, Ogden and Mayer-Davis42,Reference Vohr and McGarvey44,Reference Kramer, Hamilton and Ye46,Reference Shapira, Mandel and Mimouni53) ; or by maternal report in six studies(Reference Wallenborn, Perera and Masho39,Reference Weisband, Rausch and Kachoria40,Reference Oza-Frank, Moreland and McNamara50,Reference Oza-Frank, Chertok and Bartley56–Reference Zhao, Liu and Qiao58) . Diagnostic criteria for GDM were unclear in six studies(Reference Hummel, Vehik and Uusitalo19,Reference Dugas, Perron and Marc20,Reference Hummel, Hummel and Knopff38,Reference Chertok and Sherby47,Reference Dritsakou, Liosis and Valsami48,Reference Finkelstein, Keely and Feig59) .
No study had low risk of bias for all domains (Table 2). Only seven studies adjusted for potential confounding(Reference Hummel, Vehik and Uusitalo19,Reference Wallenborn, Perera and Masho39,Reference Weisband, Rausch and Kachoria40,Reference Oza-Frank, Moreland and McNamara50,Reference Nguyen, Binns and Lee52,Reference Uebel, Pusch and Gedrich54,Reference Finkelstein, Keely and Feig59) and nine studies were at high risk of bias due to possible confounding(Reference Dugas, Perron and Marc20,Reference Hummel, Hummel and Knopff38,Reference Hakanen, Saha and Salo43,Reference Krishnaveni, Hill and Leary45–Reference Dritsakou, Liosis and Valsami48,Reference Shapira, Mandel and Mimouni53,Reference Konig, Junginger and Reusch60) . Seven studies had high risk of bias relating to ascertainment of exposures(Reference Hummel, Hummel and Knopff38,Reference Wallenborn, Perera and Masho39,Reference Crume, Ogden and Mayer-Davis42,Reference Chertok and Sherby47,Reference Dritsakou, Liosis and Valsami48,Reference Oza-Frank, Moreland and McNamara50,Reference Finkelstein, Keely and Feig59) because exposures were not measured prior to outcomes of interest or were not well defined(Reference Hummel, Hummel and Knopff38,Reference Chertok and Sherby47,Reference Dritsakou, Liosis and Valsami48,Reference Finkelstein, Keely and Feig59) .
Table 2. Risk of bias assessment of included studies

Primary outcomes
None of the included studies reported on energy intake. Very low-quality evidence showed that infants who were and were not exposed to GDM had similar BMI at both 1–6 months (SMD 0·01, 95 % CI −0·04, 0·06; P = 0·69, I 2 = 81 %; two studies, 23 587 infants)(Reference Liu, Li and Sun55,Reference Konig, Junginger and Reusch60) and 7–12 months (SMD 0·04, 95 % CI −0·01, 0·10; P = 0·09, I 2 = 37 %; four studies, 22 612 infants)(Reference Vohr and McGarvey44,Reference Krishnaveni, Hill and Leary45,Reference Liu, Li and Sun55,Reference Konig, Junginger and Reusch60) (Table 3; online Supplementary Fig. S1). Data were not available for BMI in infants aged 13–24 months, nor for planned subgroup analyses. In sensitivity analyses, exclusion of two studies with high risk of bias(Reference Krishnaveni, Hill and Leary45,Reference Konig, Junginger and Reusch60) did not alter results.
Table 3. GRADE summary of quality of evidence for feeding and growth outcomes
(Odds ratios or standardised mean differences (SMD) and 95 % confidence intervals)

GDM, gestational diabetes mellitus; PRAMS, The Pregnancy Risk Assessment Monitoring System.
* No data available for energy intake; introduction of solid-semi solid or soft foods before 5 months of age; appetitive scores; abdominal circumference and fat-free/lean mass.
† Weight for length z-score used as BMI z-score not available(Reference Liu, Li and Sun55).
‡ Self-reported GDM history recalled by parents(Reference Wallenborn, Perera and Masho39,Reference Weisband, Rausch and Kachoria40,Reference Zhao, Liu and Qiao58) .
§ Unclear diagnosis of GDM(Reference Dritsakou, Liosis and Valsami48,Reference Finkelstein, Keely and Feig59) .
‖ Self-reported GDM history obtained from PRAMS questionnaire(Reference Oza-Frank, Chertok and Bartley56,Reference Oza-Frank and Gunderson57) .
¶ No data available for energy intake; appetitive score; breast milk composition; minimum diet diversity; food group frequency; macronutrient intake; fat-free/lean mass.
** Infants born to obese women with GDM compared with infants born to obese women without GDM(Reference Uebel, Pusch and Gedrich54).
*** No data available for energy intake; BMI; appetitive score; breast milk composition; minimum diet diversity; food group frequency; macronutrient intake; weight; length; abdominal circumference; head circumference; skinfold thickness; fat mass; fat-free /lean mass.
Secondary outcomes
Feeding patterns and behaviour
Very low-quality evidence showed that infants who were and were not exposed to GDM had similar rates of exclusive or predominant breast-feeding at ≥5 months of age (19·5 % v. 21·0 %, OR 0·89, 95 % CI 0·79, 1·01; P = 0·07, I 2 = 65 %; five studies, 30 799 infants)(Reference Logan, Emsley and Jeffries49,Reference Aris, Soh and Tint51,Reference Uebel, Pusch and Gedrich54,Reference Liu, Li and Sun55,Reference Zhao, Liu and Qiao58) and rates of no breast milk under 5 months of age (18·9 % v. 17 %, OR 1·00, 95 % CI 0·96, 1·03; P = 0·89, I 2 = 81 %; seven studies, 263 755 infants)(Reference Wallenborn, Perera and Masho39,Reference Logan, Emsley and Jeffries49,Reference Aris, Soh and Tint51,Reference Liu, Li and Sun55–Reference Zhao, Liu and Qiao58) (Table 3; online Supplementary Figs S2 and S3). However, infants born to mothers with GDM were more likely to receive formula milk/breast milk substitute before hospital discharge (OR 1·36, 95 % CI 1·22, 1·51; P < 0·00001, I 2 = 56 %; five studies, 29 089 infants)(Reference Weisband, Rausch and Kachoria40,Reference Chertok and Sherby47,Reference Oza-Frank, Moreland and McNamara50,Reference Nguyen, Binns and Lee52,Reference Finkelstein, Keely and Feig59) (Table 3; online Supplementary Fig. S4).
Infants who were exposed to GDM compared with those not so exposed were less likely to have continued breast-feeding at 12 months (65·2 % v. 73·7 %, OR 0·66, 95 % CI 0·51, 0·85; P = 0·002; one study, 1709 infants; low-quality evidence)(Reference Nguyen, Binns and Lee52) (Table 3; online Supplementary Fig. S5). Moreover, infants exposed to GDM compared with those not exposed to GDM had shorter duration of breast-feeding (months) (SMD −0·19, 95 % CI −0·26, −0·12; P < 0·00001, I 2 = 76 %; five studies, 9176 infants; very low-quality evidence)(Reference Hummel, Vehik and Uusitalo19,Reference Dugas, Perron and Marc20,Reference Hummel, Hummel and Knopff38,Reference Weisband, Rausch and Kachoria40,Reference Oza-Frank, Moreland and McNamara50) (Table 3; online Supplementary Fig. S6). No data were available for introduction of solid, semi-solid or soft foods before 5 months of age, nor for appetitive scores.
Nutritional intake
Very low-quality evidence showed that mature breast milk of women with GDM compared with women without GDM did not differ in total energy content, lactose and fat content but had lower protein content (SMD −0·36, 95 % CI −0·68, −0·04; P = 0·03, I 2 = 0 %; two studies, 272 infants)(Reference Dritsakou, Liosis and Valsami48,Reference Shapira, Mandel and Mimouni53) (Table 3; online Supplementary Fig. S7). No data were available for diet diversity, food group frequency and macronutrient intake.
Growth
At 1–6 months, low-quality evidence showed that infants who were exposed to GDM compared with those not exposed to GDM did not differ in weight but were shorter (SMD −0·09, 95 % CI −0·14, −0·04; P = 0·0008, I 2 = 0 %; four studies, 25 365 infants)(Reference Kramer, Hamilton and Ye46,Reference Logan, Emsley and Jeffries49,Reference Uebel, Pusch and Gedrich54,Reference Liu, Li and Sun55) (Table 3; online Supplementary Figs S8 and S9). Very low-quality evidence showed that at 1–6 months, infants who were exposed to GDM compared with those not exposed to GDM did not differ in head circumference or skinfold thickness (sum of four skinfolds)(Reference Uebel, Pusch and Gedrich54) but had greater fat mass (SMD 0·53, 95 % CI 0·13, 0·94; P = 0·010, I 2 = 0 %; two studies, ninety-seven infants)(Reference Logan, Emsley and Jeffries49,Reference Uebel, Pusch and Gedrich54) (Table 3; online Supplementary Figs S10, S12 and S13). No data were available for abdominal circumference or fat-free mass at 1–6 months of age.
At 7–12 months, low-quality evidence showed that infants who were exposed to GDM compared with those not exposed to GDM did not differ in weight but were again shorter (SMD −0·07, 95 % CI −0·13, −0·02; P = 0·005, I 2 = 0 %; four studies, 25 736 infants)(Reference Vohr and McGarvey44,Reference Krishnaveni, Hill and Leary45,Reference Uebel, Pusch and Gedrich54,Reference Liu, Li and Sun55) (Table 3; online Supplementary Figs S8 and S9). Very low-quality evidence showed that at 7–12 months, infants who were exposed to GDM compared with those not exposed to GDM did not differ in abdominal circumference(Reference Vohr and McGarvey44,Reference Uebel, Pusch and Gedrich54) , head circumference, skinfold thickness (sum of four skinfolds)(Reference Vohr and McGarvey44,Reference Uebel, Pusch and Gedrich54) , triceps skinfold(Reference Krishnaveni, Hill and Leary45), subscapular skinfold(Reference Krishnaveni, Hill and Leary45) and fat mass(Reference Uebel, Pusch and Gedrich54) (Table 3; online Supplementary Figs S10, S11, S12 and S13). No data were available for fat-free mass at 7–12 months or for any growth outcomes at 13–24 months of age.
Studies not included in quantitative synthesis
In the EPOCH study, GDM was not associated with altered mean infant BMI or BMI growth trajectory from birth to 26 months (n 504)(Reference Crume, Ogden and Daniels41), and rates of adequate breast-feeding, that is, breast-feeding ≥ 6 months (44 % v. 47 %, n 493; P = 0·54)(Reference Crume, Ogden and Mayer-Davis42). This study had uncertain to high risk of bias (Table 2). A Finnish study (n 6609) found no difference in mean peak BMI between infants who were and were not exposed to GDM, although infant BMI peaked slightly earlier in those exposed to GDM (9·9 v. 10·4 months; P = 0·05)(Reference Hakanen, Saha and Salo43). The study had high risk of bias for potential confounding (Table 2). A Chinese study found that among boys who were born with appropriate birth weight for gestation, those exposed to GDM compared with those who were not so exposed had less gain in weight and length from 3 to 6 months of age (mean weight 1. 1 (sd 0·4) v. 1. 4 (sd 0·4) kg, P = 0·040; length 4·9 (sd 2·3) v. 6·3 (sd 1·2) cm, P = 0·026), but not from birth to 3 months or from 6 to 13 months. No differences in growth were seen in girls who were and were not exposed to GDM. The study had uncertain risk of bias (Table 2)(Reference Zhao, Ma and Zhang37).
Discussion
We found low- to very low-quality evidence that the infants exposed to GDM, compared with infants not exposed, had similar BMI and weight to 12 months of age but were slightly shorter (about 0·5 cm) at 1–6 and 7–12 months. Nevertheless, infants exposed to GDM had increased total fat mass at 1–6 months (about 200 g) but not at 7–12 months. Subcutaneous fat, as measured by skinfold thickness, also did not differ in the first year. With regard to feeding, we found low to very low-quality evidence that infants exposed to GDM, compared with control infants, were about 40 % more likely to receive formula milk/breast milk substitute before hospital discharge, about 30 % less likely to have continued breast-feeding at 12 months and had about 1 month shorter mean duration of breast-feeding. Further, breast milk of women with GDM compared with women without GDM was similar in energy, fat and carbohydrate content but had about 4 g/l lower protein concentration. No data were available on energy intake, complementary feeding, infant appetitive traits and nutritional intake.
Rapid weight gain during early infancy, especially in fat mass, is associated with increased risk of childhood obesity(Reference Scheurer, Zhang and Gray69,Reference Koontz, Gunzler and Presley70) . Thus, our finding that infants born to women with GDM had increased whole-body fat mass at 3–4 months provide one possible explanation for the association between GDM and childhood obesity. An increase in fat mass of ≥ 200 g represents 75 % of the average monthly gain in fat mass at 3–6 months and is likely to be clinically important(Reference Koontz, Gunzler and Presley70,Reference Eriksson, Lof and Forsum71) . For example, in a mixed population of infants, 38 % of whom were exposed to GDM, the odds of overweight or obesity in mid-childhood was increased 8-fold for every additional 100 g in fat mass gained per month up to 8 months(Reference Koontz, Gunzler and Presley70). The fact that weight and BMI were similar between infants who were and were not exposed to GDM suggests that the accelerated gain in fat mass is associated with slower growth in fat-free mass. Further, given similar skinfold thickness between exposure groups, the increased fat deposition may be intra-abdominal, which in adolescents and adults is associated with greater risk of cardio-metabolic disease, especially type 2 diabetes(Reference Lee, Pedley and Hoffmann72–Reference Goran and Gower74). Although fat mass was similar between exposure groups at 7–12 months, this does not preclude future effects of GDM on offspring body composition, as several longitudinal cohorts have shown that BMI trajectories of GDM and non-GDM cohorts converge about 12 months and only to separate again after 6 years of age(Reference Crume, Ogden and Daniels41,Reference Silverman, Rizzo and Cho75,Reference Crume, Ogden and West76) .
One potential pathway for the increased fat gain in early infancy associated with GDM is reduced breast-feeding. WHO recommends initiation of breast-feeding within 1 h of birth, exclusive breast-feeding up to 6 months of age and continuation of breast-feeding along with complementary foods until at least 24 months of age(77–Reference Owen, Whincup and Cook79). Importantly, breast-feeding, compared with formula feeding, is associated with reduced risk of childhood obesity and metabolic disorders in adult life(Reference Arenz, Ruckerl and Koletzko10). This may be related to lower overall energy intake in breastfed infants(Reference Butte, Wong and Hopkinson80), or the influence of breast milk hormones on appetitive traits(Reference Fahrenkrog, Harder and Stolaczyk17,Reference Young, Levek and Reynolds81) and growth. Although we did not find that GDM was associated with reduced exclusive/predominant breast-feeding in the first 5 months, overall duration of breast-feeding was reduced and use of formula in hospital was substantially increased. The latter may be an important risk factor, as even brief supplementation with formula or protein in preterm infants has been associated with increased risk of later obesity(Reference Singhal, Kennedy and Lanigan14). It is interesting that breast milk of women with GDM had lower protein content, which might be expected to be protective against excess adiposity in infancy and obesity in childhood(Reference Koletzko, von Kries and Closa82,Reference Weber, Grote and Closa-Monasterolo83) , although some studies in preterm infants have shown that lower protein intake is associated with a transient increase in fat mass in early infancy(Reference Roggero, Gianni and Amato84).
Although the effect size was small, a consistent finding in infants exposed to GDM at both the 1–6 and 7–12 months epochs was shorter length. The reasons for and long-term significance of this is unclear. It is evident that insulin is important for bone and muscle growth(Reference Oldknow, MacRae and Farquharson85–Reference Davis87). However, it has also been demonstrated that preterm infants exposed to higher concentrations of insulin have shorter leg length at term corrected age and reduced stature at mid-childhood(Reference Alsweiler, Harding and Bloomfield88,Reference Tottman, Alsweiler and Bloomfield89) . A similar response may occur with fetal hyperinsulinism in GDM.
Limitations
A key limitation of this systematic review is a lack of high-quality data. This was primarily due studies being observational with several at high risk of bias due to confounding and ascertainment of exposures. The quality of evidence was also limited by imprecise estimates and heterogeneity. Moreover, no data were available for several outcomes, including the primary outcome of energy intake. Given the importance of early nutrition for long-term metabolic health(Reference Rolland-Cachera, Akrout and Péneau90), it is surprising that no studies have assessed the effect of GDM on infant energy intake, nutrition, complementary feeding and appetitive traits. The latter may be particularly important in explaining associations between GDM and obesity in offspring. For example, in animals, consumption of breast milk from GDM mothers affects satiety centres in the infant brain(Reference Fahrenkrog, Harder and Stolaczyk17), leading to consumption of larger and more frequent feeds, and increased energy intake, thereby increasing the risk of obesity. Additionally, for some outcomes, there was insufficient information to draw any conclusion due to inadequate sample size. Thus, results of this review must be interpreted cautiously, and higher-quality evidence from large well-designed prospective cohort studies is needed.
Another limitation is that studies included in this review provided few data on maternal treatment of GDM and the degree of glycaemic control that was achieved. Only six studies provided data on the proportion of women treated with either diet or with medications, such as insulin, metformin or sulphonylureas(Reference Krishnaveni, Hill and Leary45,Reference Kramer, Hamilton and Ye46,Reference Logan, Emsley and Jeffries49,Reference Shapira, Mandel and Mimouni53,Reference Uebel, Pusch and Gedrich54,Reference Konig, Junginger and Reusch60) . Variations in treatment of GDM may have contributed to the substantial heterogeneity seen for several outcomes, as infants whose mothers have well controlled v. poorly controlled GDM are likely to be different. The varying approaches to screening and diagnosis of GDM may have also contributed to heterogeneity, as women meeting different diagnostic thresholds are known to be at different risk for perinatal complications(Reference Saccone, Caissutti and Khalifeh91). We planned to explore the effect of higher and lower degrees of maternal dysglycaemia on infant outcomes, including whether women met higher or lower glycaemic thresholds for diagnosis, were treated or untreated, or had tighter or less tight glucose control, but data were unavailable for this pre-specified subgroup analysis.
The association between GDM and infant feeding and growth outcomes may be confounded by maternal BMI, as larger maternal size is a risk factor for both GDM and offspring obesity(Reference Drake and Reynolds92). We planned to use adjusted estimates of exposure effect in meta-analysis, but only two studies included maternal BMI as a covariate in regression analysis. Thus, this remains a potential source of bias that should be addressed in future studies. Similarly, there is some evidence to suggest that management of GDM with metformin compared with insulin may be associated with greater gains in subcutaneous fat in early childhood(Reference Rowan, Rush and Obolonkin93,Reference Okesene-Gafa, Li and McKinlay94) and it will be important that future studies explore the effect of maternal mode of treatment on infant growth outcomes.
Recommendations for research
Given that the population of infants exposed to GDM is continuously on the rise, it is important that the effects of GDM on infant growth, nutrition and feeding, and underlying mechanisms, are elucidated. This review has outlined key infant outcomes and mechanistic pathways that should be evaluated in large prospective studies, including potentially modifiable factors, such as breast-feeding, use of formula in hospital and complementary feeding. Nested cohort studies within relevant clinical trials are the most suitable design to provide the highest quality evidence for the effect of GDM on infant outcomes. For example, clinical trials of GDM screening and diagnosis provide an opportunity to prospectively evaluate infant outcomes after different degrees of maternal dysglycaemia, with adjustment for potential confounding, including maternal BMI, age and socio-economic status. One such large study is currently underway (ACTRN12615000290594). It is also important that infant outcomes are fully assessed in GDM treatment trials to investigate the extent to which any adverse effects of GDM on offspring are preventable.
Conclusions
There was low- to very low-quality evidence that infants exposed to GDM, compared with those not exposed, have similar BMI from 1 to 12 months of age. No data were reported on energy intake. However, infants exposed to GDM had reduced length at 1–6 and 7–12 months, increase whole-body fat at 1–6 months, higher rates of formula supplementation in hospital, shorter duration of breast-feeding and decreased rates of continued breast-feeding at 12 months. Breast milk of women with GDM had lower protein content. There was no association between GDM and infant weight, skinfold thickness at 1–6 and 7–12 months. No data were available to assess the effect of GDM on macronutrient intake, diet quality, complementary feeding, appetitive traits, fat-free mass or outcomes at 13–24 months. Large, well-designed prospective cohort studies are needed to determine if the association between GDM and later risk of obesity and diabetes is mediated by altered infant feeding, nutrition and growth. This is an important knowledge gap that must be addressed if effective strategies are to be found to reverse intergenerational risks of obesity and type 2 diabetes mellitus related to GDM.
Acknowledgements
This review was unfunded.
K. M. drafted the protocol, conducted the literature search, performed hand-searching to identify additional studies, screened title, abstracts and full-texts, extracted data, assessed risk of bias and quality of evidence, analysed data and interpreted results and drafted the manuscript. C. M. contributed to the protocol, assessed full-text eligibility, extracted data, assessed risk of bias and quality of evidence, assisted in analysis and interpretation of data and assisted with drafting of the manuscript. J. H. contributed to the protocol, assessed full-text eligibility, extracted the data, assessed the risk of bias and critically reviewed the manuscript. C. C. contributed to the protocol, assessed full text eligibility and critically reviewed the manuscript.
The authors declare that there are no conflicts of interest.
Supplementary material
For supplementary materials referred to in this article, please visit https://doi.org/10.1017/S0007114520000264