The regulations of feed intake and whole-body energy homoeostasis are particularly important for grazing ruminants in pastures where nutrient and energy intakes can fluctuate dramatically with season and often are below maintenance requirements(Reference Roche, Blache and Kay1). The regulation of whole-body energy homoeostasis is a complex process and involves multiple tissues such as adipose and liver, and multiple systems, such as the endocrine and nervous systems. The hypothalamus undertakes the role of regulating whole-body energy homoeostasis by integrating signals received on the body energy status and then mediating nutrients intake, body reserves and energy expenditure(Reference Roche, Blache and Kay1,Reference Loh, Herzog and Shi2) . This function of the hypothalamus has been identified in rodents, as well as in domestic animals(Reference Roche, Blache and Kay1–Reference Mühlhäusler, McMillen and Rouzaud5).
Within the arcuate nucleus (ARC) of the hypothalamus, two distinct populations of neurons exist that have major roles in modulating energy homoeostasis: one population co-expresses the orexigenic neuropeptide Y (NPY)/agouti-related protein (AgRP), and the other co-expresses anorexigenic proopiomelanocortin (POMC)/cocaine and amphetamine-regulated transcript (CART)(Reference Loh, Herzog and Shi2,Reference Hahn, Breininger and Baskin6) . Owing to the semi-permeable blood–brain barrier, these two populations of neurons have direct access to each other and respond rapidly to peripheral signals, such as insulin, leptin and gut peptides, including ghrelin, glucagon-like peptide-1 (GLP-1), glucose-dependent insulinotropic polypeptide (GIP) and peptide YY(Reference Loh, Herzog and Shi2,Reference Murphy and Bloom4,Reference Sainsbury and Zhang7) . The hypothalamus integrates these signals and modifies the expression of neuropeptides of NPY/AgRP or POMC/CART, which affect energy homoeostasis by regulating energy intake and expenditure(Reference Murphy, Dhillo and Bloom8–Reference Stanley, Wynne and McGowan11). Furthermore, hypothalamic adenosine monophosphate-activated protein kinase (AMPK) plays a crucial role in energy balance by increasing energy production and reducing energy consumption. The AMPK provides a link between peripheral signals and central nervous pathways and is essential for the regulation of the expression of orexigenic and anorexigenic neuropeptides(Reference Claret, Smith and Batterham12–Reference Oh, Cho and Cho15).
Tibetan sheep (Ovis aries), an indigenous breed raised at altitudes above 3000 m on the Qinghai-Tibetan Plateau (QTP), play vital roles in the livelihood of Tibetan pastoralists and in the QTP ecosystem(Reference Miller16,Reference Shang, Gibb and Leiber17) . Traditionally, these sheep only graze natural pasture all year round. Their energy intake fluctuates greatly among seasons and can be extremely low for extended periods in winter, when they commonly lose body weight (BW) and are in negative energy balance(Reference Long, Apori and Castro18,Reference Ding, Shi and Zhang19) . However, they have adapted well to the harsh conditions of the QTP, being able to cope with low energy intake and large BW losses in the extreme winters. Therefore, energy homoeostasis regulation is important in the adaptation of Tibetan sheep to the harsh QTP, and a better understanding of energy homoeostasis regulation would be important in improving their husbandry.
Little information is available on how the hypothalamic pathways regulate whole-body energy homoeostasis of Tibetan sheep. The aim of this study was to fill this gap by comparing Tibetan and Small-tailed Han sheep. Small-tailed Han sheep (Ovis aries) were introduced to the QTP due to their high lamb production, are raised in feedlots and graze natural pasture only in summer when the forage is of good quality(Reference Miao and Luo20). In earlier companion studies on the same sheep, Tibetan sheep had lower maintenance energy requirements and greater average daily gain than Small-tailed Han sheep when consuming the same diet(Reference Jing, Zhou and Wang21). In addition, Tibetan sheep had a greater capacity to absorb SCFA(Reference Jing, Wang and Degen22) and a more flexible capacity in glucose and fatty acid metabolism in adipose tissue(Reference Jing, Zhou and Degen23). Based on the different backgrounds between breeds and on our previous studies, we hypothesised that Tibetan and Small-tailed Han sheep would differ in their hypothalamus pathways to modulate energy homoeostasis of the whole body and predicted that the differences would allow Tibetan sheep to cope better with low energy intake than Small-tailed Han sheep. To test this hypothesis, we compared the key expressions of regulators in the hypothalamic NPY/AgRP and POMC/CART pathways between breeds when consuming diets of different energy concentrations.
Materials and methods
This study was approved by the Institutional Animal Care and Use Committee of Lanzhou University (SCXK Gan 20140215) and conducted at the Yak and Tibetan Sheep Research Station of Lanzhou University, Tianzhu Tibetan Autonomous County, Gansu Province, north-eastern QTP, China.
Animals and experiment design
Details on animals and diets were described previously in a companion study on the same sheep(Reference Jing, Zhou and Wang21). Briefly, twenty-four Tibetan sheep (BW = 48·5 ± 1·89 kg) and twenty-four Small-tailed Han sheep (BW = 49·2 ± 2·21 kg), all wethers aged 1·5 years, were housed individually under a three-sided roofed shelter and were allocated randomly into one of four groups (6 sheep/group per breed). Each group was offered a diet of different digestible energy density: 8·21, 9·33, 10·45 or 11·57 MJ/kg DM (online Supplementary Table S1). The diets all contained ∼70 g/kg crude protein, which was similar to the average crude protein content in forage of the QTP during the cold season(Reference Xie, Chai and Wang27). The diet, at 4·5 % BW0·75 DM per day, was offered twice daily (08.00 and at 18.00 hours) in approximately two equal portions and intake was measured daily. It was designed that the diets would provide 0·8, 0·9, 1·0 and 1·1 times maintenance energy requirements (Feeding Standards of Meat Producing Sheep and Goats of China, NY/T 816-2004)(28). Drinking water was available ad libitum. Sheep were weighed before morning feeding every 2 weeks to calculate average daily gain, and it was assumed that a change in BW reflected a change in energy balance in the same direction. Nutrients intakes and average daily gain of these sheep were reported earlier(Reference Jing, Zhou and Wang21). All sheep were slaughtered humanely 2–3 h after the morning feeding on day 49.
Blood sample collection and gut hormones determination
Jugular blood samples (15 ml) were collected on day 42 for determination of hormone concentrations. The samples were taken from both breeds on the four dietary treatments, 30 min before morning feeding. A daily rhythm of hormone concentrations based on feeding schedule was reported, with a transient surge of plasma ghrelin in the pre-feeding period in ruminants on a scheduled feeding plan(Reference Sugino, Hasegawa and Kikkawa24–Reference Miura, Hojo and Takahashi26). From these findings, one can infer that blood hormonal measurements can be compared between breeds if samples are collected under the same conditions at a set time before a scheduled feeding. This was the case in the present study – blood samples were collected 30 min before morning feeding in all animals.
The samples were kept on ice immediately after collection and centrifuged at 2000 × g for 15 min at 4°C. The serum was snap-frozen in liquid N2 and then stored at −80°C until assayed. The concentrations of NPY, ghrelin, GLP-1 and GIP were determined within 2 weeks using commercial ELISA kits (Ovis, Shanghai Bangyi Bioscience Co., Ltd) by a plate reader (352, LabSystems Multiskan MS, Thermo Fisher Scientific Inc) according to the manufacturer’s instructions. The concentration of active ghrelin was measured with 4-(2-aminoethyl) benzenesulfonyl fluoride hydrochloride to prevent deacylation. The sensitivity of the assay was 1 pg/ml, and the intra- and inter-assay variations were 4·3 and 4·6 %, respectively.
RNA extraction and real-time quantitative PCR
The brain was removed immediately after slaughter and placed on ice. Tissue samples from the ARC of the hypothalamus were collected as described by Selehi et al. (Reference Salehi, Namavar and Shirazi29) and David et al. (Reference David Glass, Amann and Nett30). Briefly, the ARC is located at the base of the hypothalamus on either side of the third cerebroventricle. The diencephalon was dissected out by a coronal section anterior to the optic chiasm, and coronal cut was made at the posterior border of the mammillary bodies. To separate the ARC, a third coronal cut was made through the middle of the optic tract, just rostral to the infundibulum. The excised tissue was snap-frozen in liquid N2 and stored at –80°C until assayed. The process from slaughter till freezing of sample was up to 20 min. The tissues were crushed to a fine powder in a mortar with liquid N2; total RNA was isolated using Trizol reagent (Invitrogen, Life Technologies Waltham), and, then, qualified RNA (checked by agarose gel electrophoresis and spectrophotometrically) was reverse-transcribed to cDNA by Prime Script RT Reagent Kit (Takara Biotechnology Co., Ltd) according to the manufacturer’s instructions. The cDNA was amplified by real-time PCR with the Agilent StrataGene Mx3000P (Agilent Technologies Inc) using an SYBR Green real-time PCR master mix kit (Takara). The amplification efficiency for each gene was calculated, and melting curve analysis and gel electrophoresis verified that the expected target was amplified. Primers of NPY, neuropeptide Y Y1 receptor (NPY1R), neuropeptide Y Y5 receptor (NPY5R), AgRP, POMC, melanocortin 3 receptor (MC3R), melanocortin 4 receptor (MC4R), CART, glucagon-like peptide-1 receptor, glucose-dependent insulinotropic polypeptide receptor (GIPR), insulin receptor, leptin receptor, adenosine monophosphate-activated protein kinase-α 2 (AMPKα2) and β-Actin are presented in online Supplementary Table S2. The β-Actin was selected as a reference gene as its expression was stable in different groups. Relative gene mRNA expression levels were quantified according to the 2–ΔΔCt method(Reference Livak and Schmittgen31).
Protein extraction and western blotting
Total proteins were extracted from the hypothalamic ARC using a tissue protein extraction reagent (MDL91201, MDL Biotech Co., Ltd), and the protein concentration was determined using a protein assay kit (MDL9135053, MDL Biotech Co., Ltd). Then, equal amounts of protein were separated by SDS-PAGE, blotted to polyvinylidene fluoride membranes (Millipore, ISEQ00010), blocked with blocking reagent (MDL912056, MDL Biotech Co., Ltd), immunoblotted with the indicated antibodies and visualised using enhanced chemiluminescence (Beyotime Biotechnology Inc.), as described by Dessauge et al. (Reference Dessauge, Lollivier and Ponchon32) and Börner et al. (Reference Börner, Albrecht and Schäff33). The membranes were exposed to the chemiluminescence imaging system (ChemiDoc MP 170–8280, Bio-rad), and the integrated optical density of bands was quantified using ImageJ 1.52d software. Each sample was normalised to β-Actin content. The antibody information and dilutions were as follows: primary antibodies against NPY (1:100, sc-133080 Santa Cruz Biotechnology), NPY1R (1:100, sc-393192 Santa Cruz Biotechnology), NPY5R (1:100, sc-137167 Santa Cruz Biotechnology), AgRP (1:100, BYK-7376R Boyan Biotechnology Co., Ltd), POMC (1:200, sc-57021 Santa Cruz Biotechnology), MC3R (1:500, bs-7558R Bioss Antibodies Inc), MC4R (1:100, sc-55567, Santa Cruz Biotechnology), CART (1:500, bs-12145R Bioss Antibodies Inc), glucagon-like peptide-1 receptor (1:100, sc-390773, Santa Cruz Biotechnology), GIPR (1:500, bs-13292R Bioss Antibodies Inc), insulin receptor (1:100, sc-57344 Santa Cruz Biotechnology), leptin receptor (1:100, sc-8391, Santa Cruz Biotechnology), AMPKα2 (1:100, sc-74461 Santa Cruz Biotechnology) and β-Actin (1:500, MD-6553 MDL biotech Co., Ltd), and the corresponding secondary antibodies (goat anti-rabbit IgG HRP sc-2004, rabbit anti-mouse IgG HRP sc-358914, Santa Cruz Biotechnology).
Statistical analysis
Data were analysed using the mixed model of the SAS statistical package (SAS version 9.4, SAS Inst. Inc.) as: Y = µ + E + B + (E × B) + e, where Y is the dependent variable, µ is the overall mean, E is the effect of dietary energy concentration, B is the effect of sheep breed, E × B is the interaction between sheep breed and dietary energy concentration and e is the residual error. Sheep breed and dietary energy concentration were fixed effects, and the experimental animals were random effects. When there was a significant interaction between dietary energy concentration and breed, differences between breeds at the same dietary energy concentration were separated using t tests(Reference Zhou, Guo and Kang34). Differences were considered significant at P < 0·05.
Results
Concentrations of brain–gut peptide in serum
Tibetan sheep had a greater concentration of serum ghrelin at the two lowest dietary energy concentrations but a lesser concentration at the highest energy concentration than Small-tailed Han sheep (dietary energy concentration × breed, P < 0·001; Fig. 1). Tibetan sheep also had greater serum concentrations of GIP and GLP-1 than Small-tailed Han sheep (breed, P < 0·01; Fig. 1). The serum concentration of NPY was greater in Tibetan sheep at the lowest dietary energy concentration but lesser at the two highest energy concentrations than Small-tailed Han sheep (dietary energy concentration × breed, P < 0·001; Fig. 1).
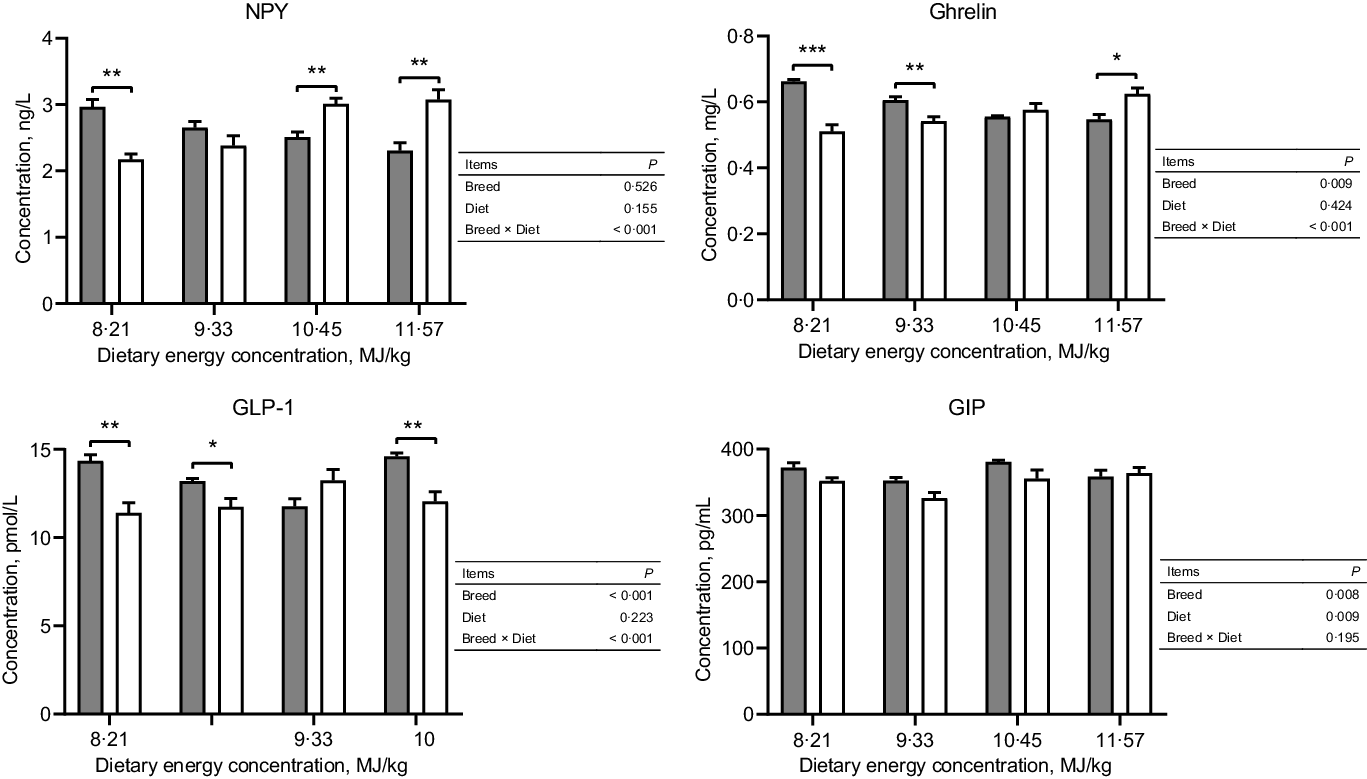
Fig. 1. Serum concentrations of NPY and gut peptides of Tibetan and Small-tailed Han sheep offered diets of different energy concentrations. The dietary energy concentrations are digestible energy on a DM basis. NPY, neuropeptide Y; GIP; glucose-dependent insulinotropic polypeptide; GLP-1; glucagon-like peptide-1. Values are means with their standard error of the means. *P < 0·05; **P < 0·01; ***P < 0·001. , Tibetan sheep;
, Small-tailed Han heep.
Expressions of hormone and gut peptide receptors in the hypothalamus
The expressions of hormone and gut peptide receptors in the ARC of the hypothalamus are presented in Figs. 2 and 3. The mRNA expression of GIPR was greater in Small-tailed Han than Tibetan sheep at the two highest energy concentrations (dietary energy concentration × breed, P = 0·012), but the GIPR protein expression was greater in Tibetan than Small-tailed Han sheep (P < 0·001; Fig. 2). Tibetan sheep also had greater mRNA and protein expressions of glucagon-like peptide-1 receptor than Small-tailed Han sheep (P < 0·01; Fig. 2). Small-tailed Han sheep had a greater leptin receptor mRNA expression (P < 0·001) but lesser protein expression than Tibetan sheep (P < 0·001; Fig. 3). Moreover, Tibetan sheep had greater mRNA and protein expression of IR than Small-tailed Han sheep (P < 0·001; Fig. 3).
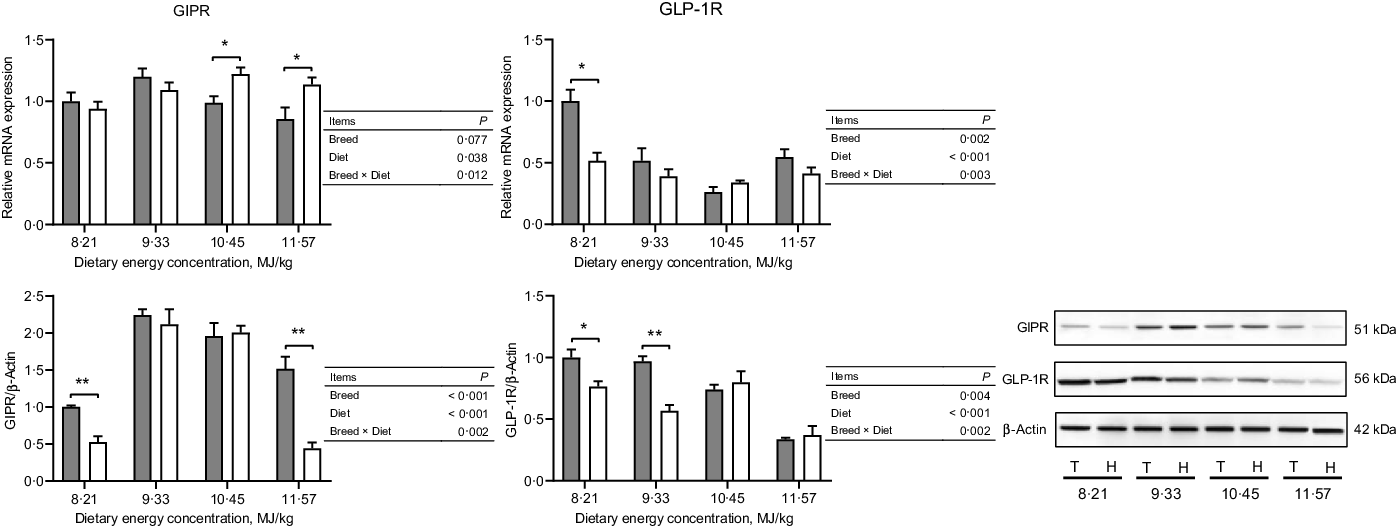
Fig. 2. The expressions of gut peptide receptors in the hypothalamus of Tibetan (T) and Small-tailed Han (H) sheep offered diets of different energy concentrations. The dietary energy concentrations are digestible energy on a DM basis. GIPR, glucose-dependent insulinotropic polypeptide receptor; GLP-1R, glucagon-like peptide-1 receptor. Values are means with their standard error of the means. *P < 0·05; **P < 0·01. , Tibetan sheep;
, Small-tailed Han sheep.
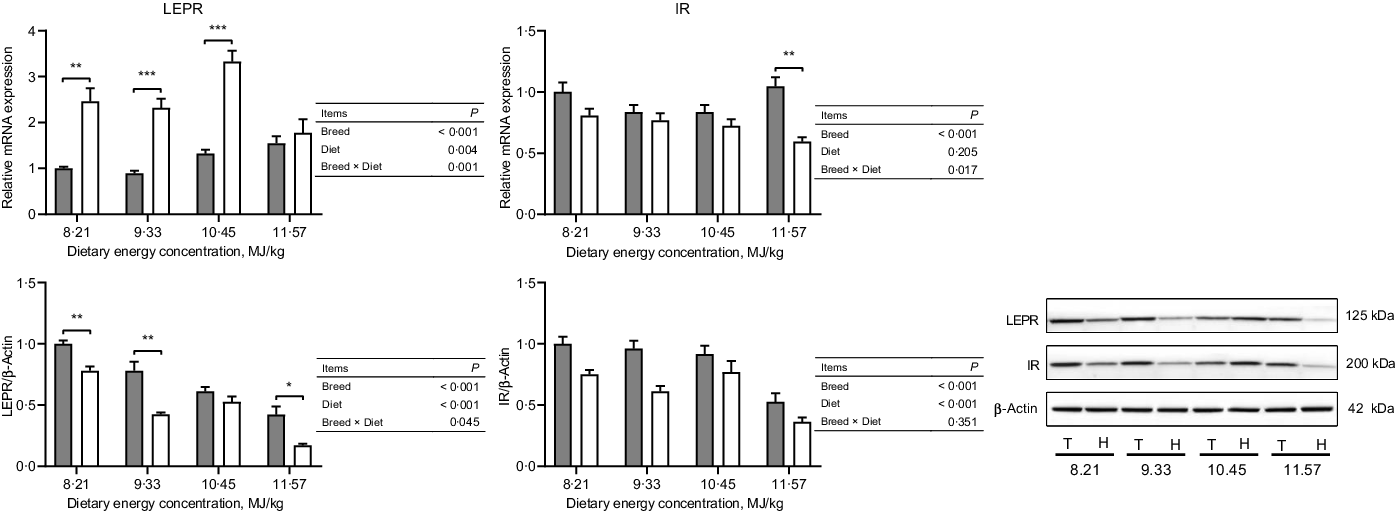
Fig. 3. The expressions of hormone receptors in the hypothalamus of Tibetan (T) and Small-tailed Han (H) sheep offered diets of different energy concentrations. The dietary energy concentrations are digestible energy on a DM basis. InsR, insulin receptor; LEPR, leptin receptor. Values are means with their standard error of the means. *P < 0·05; **P < 0·01; ***P < 0·001. , Tibetan sheep;
, Small-tailed Han sheep.
Expressions of neuropeptides of energy homoeostasis regulation in the hypothalamus
In the NPY/AgRP regulation pathway, Small-tailed Han sheep had greater mRNA expressions of NPY, NPY1R and NPY5R (P < 0·001) but lesser protein expressions than Tibetan sheep at the two lowest dietary energy concentrations in the ARC of the hypothalamus (dietary energy concentration × breed, P < 0·01; Fig. 4). The AgRP mRNA expression was greater (P < 0·001), but the protein expression was lesser (P = 0·002) in Small-tailed Han than Tibetan sheep (Fig. 4). However, in the POMC/CART regulation pathway, the mRNA expression of POMC in the ARC of the hypothalamus was greater in Small-tailed Han than Tibetan sheep (P < 0·001), and the POMC protein expression was greater in Small-tailed Han sheep at the two highest dietary energy concentrations (dietary energy concentration × breed, P = 0·001; Fig. 5). The POMC receptors of MC3R and MC4R mRNA and protein expressions were greater in Small-tailed Han than Tibetan sheep (P < 0·01; Fig. 5), which was consistent with the greater POMC mRNA expression in Small-tailed Han than Tibetan sheep. The CART mRNA expression was greater in Tibetan sheep at the two lowest dietary energy concentrations but lesser at the highest dietary energy concentration than Small-tailed Han sheep (dietary energy concentration × breed, P < 0·001); however, the CART protein expression was greater in Small-tailed Han than Tibetan sheep (P = 0·003; Fig. 5). In the AMPK regulation pathway, Small-tailed Han sheep had a greater AMPKα2 mRNA expression (P = 0·008) but lesser AMPKα2 protein expression than Tibetan sheep (P < 0·001; Fig. 6).

Fig. 4. The expressions of NPY/AgRP pathway in the hypothalamus of Tibetan (T) and Small-tailed Han (H) sheep offered diets of different energy concentrations. The dietary energy concentrations are digestible energy on a DM basis. AgRP, agouti-related peptide; NPY, neuropeptide Y; NPY1R, neuropeptide Y Y1 receptor; NPY5R, neuropeptide Y Y5 receptor. Values are means with their standard error of the means. *P < 0·05; **P < 0·01; ***P < 0·001. , Tibetan sheep;
, Small-tailed Han sheep.

Fig. 5. The expressions of POMC/CART pathway in the hypothalamus of Tibetan (T) and Small-tailed Han (H) sheep offered diets of different energy concentrations. The dietary energy concentrations are digestible energy on a DM basis. CART, cocaine and amphetamine-regulated transcript; MC3R, melanocortin 3 receptor; MC4R, melanocortin 4 receptor; POMC, proopiomelanocortin. Values are means with their standard error of the means. *P < 0·05; **P < 0·01; ***P < 0·001. , Tibetan sheep;
, Small-tailed Han sheep.

Fig. 6. The expression of AMPKα
2 in the hypothalamus of Tibetan (T) and Small-tailed Han (H) sheep offered diets of different energy concentrations. The dietary energy concentrations are digestible energy on a DM basis. AMPKα2, adenosine monophosphate-activated protein kinase-α 2. Values are means with their standard error of the means. *P < 0·05; **P < 0·01. , Tibetan sheep;
, Small-tailed Han sheep.
Discussion
Energy homoeostasis is affected by feed consumption, energy metabolism and the amount of energy allotted for production. Therefore, a better understanding of the regulatory mechanisms of whole-body energy homoeostasis in sheep is important in improving their production. Tibetan sheep are late maturing and reach puberty between 10 and 18 months of age. To avoid the effect of age of sheep in the present study, as age is an important factor in the development of both the central and peripheral homoeostasis regulatory systems(Reference Yang, Tien and Boddupalli35–Reference Huang, Lu and Xi37), mature (18 months of age) Tibetan and Small-tailed Han sheep were used. Additionally, DM intake is an important factor in the regulation of energy homoeostasis, specifically, in stimulating the secretion of gastrointestinal peptide(Reference Roche, Blache and Kay1,Reference Relling and Reynolds38,Reference Relling, Pate and Reynolds39) . In this study, we were interested in the effect of energy intake on the hypothalamic regulation of energy homoeostasis in the sheep. To avoid the effect of DM intake, each group received the same amount of feed and protein per day, but with different energy yields. As designed, sheep were either near maintenance, below maintenance or above maintenance energy requirements with the same DM intake. The group at near maintenance (zero change in BW) acted as controls for the other three groups. The BW increased linearly with an increase in dietary energy concentration, and average daily gain was greater in Tibetan than Small-tailed Han sheep when consuming the same DM and energy intake. Tibetan sheep gained BW and were assumed to be in positive energy balance at dietary energy levels of 9·33, 10·45 and 11·57 MJ/kg, and lost BW and were in negative energy at dietary energy level of 8·21 MJ/kg, whereas Small-tailed Han sheep gained BW and were in positive energy balance at dietary energy levels of 10·45 and 11·57 MJ/kg, and lost BW and were in negative energy balance at the two lowest dietary energy levels of 8·21 and 9·33 MJ/kg (online Supplementary Table S3)(Reference Jing, Zhou and Wang21). Consequently, Tibetan sheep were able to maintain energy balance at a lower energy intake and their energy requirements for maintenance were lower than for Small-tailed Han sheep. The hypothalamus plays a key role in regulating energy homoeostasis of the whole body through integrating signals on energy status from the periphery. This study supported our prediction that the hypothalamic pathways of Tibetan sheep would possess a better capability to modulate energy homoeostasis of the whole-body than Small-tailed Han sheep.
The statuses of energy intake, energy metabolism and body energy reserves are integrated in the hypothalamic ARC nucleus, which then modulates energy homoeostasis through orexigenic NPY/AgRP- and anorexigenic POMC/CART-containing neurons(Reference Sartin, Daniel and Whitlock3,Reference Schwartz, Woods and Porte9) . NPY and AgRP neuropeptides are important potent agents for net energy gain by simultaneously increasing energy intake and decreasing energy expenditure(Reference Loh, Herzog and Shi2,Reference Miner, Della-Fera and Paterson40–Reference Kalra, Dube and Sahu42) . The hypothalamic NPY protein expression and circulating level increase when in negative energy balance and decrease when in positive energy balance, but the opposite pattern occurs in the hypothalamic POMC and CART expressions(Reference Archer, Rhind and Findlay43–Reference Kim, O’Hare and Grace45), which were consistent with the results of both Tibetan and Small-tailed Han sheep in the present study. Furthermore, NPY derived from the ARC decreases brown adipose tissue thermogenesis and reduces energy expenditure(Reference Shi, Lau and Lin46). In the present study, DM intake was the same for the different dietary energy concentrations and between breeds(Reference Jing, Zhou and Wang21). However, when in negative energy balance, the greater NPY serum concentration and protein expression in the ARC in Tibetan sheep indicated a lower energy expenditure than in Small-tailed Han sheep, which was consistent with the lower BW loss and lower maintenance energy requirements in Tibetan than in Small-tailed Han sheep(Reference Jing, Zhou and Wang21). The long-term adaptation of Tibetan sheep to low energy intake and low temperature in the QTP could explain, at least in part, these results. In contrast, when in positive energy balance, Small-tailed Han sheep had higher NPY concentration and protein expression than Tibetan sheep, which may be linked to the high-energy diets and intensive feeding of Han sheep. The regulation of energy homoeostasis in the NPY pathway involves multiple receptor subtypes, with the Y1 and Y5 isoforms (NPY1R and NPY5R) most strongly implicated(Reference Kanatani, Ito and Ishihara47–Reference Nguyen, Mitchell and Lin50). Therefore, the greater protein expressions of NPY1R and NPY5R in Tibetan than in Small-tailed Han sheep were consistent with the greater NPY protein expression in Tibetan sheep when in negative energy balance. These responses were reversed when in positive energy balance. However, the greater mRNA expressions of NPY, AgRP, NPY1R and NPY5R did not lead to a greater expression of proteins in Small-tailed Han than in Tibetan sheep when in negative energy balance, which suggested that Tibetan sheep, most likely, had a greater capacity of transcription regulation in the NPY/AgRP pathway protein expression. Activation of POMC/CART neurons releases POMC and CART, which exerts an inhibitory action on feed intake and energy storage but enhances energy expenditure through two hypothalamic receptors, MC3R and MC4R(Reference Sartin, Daniel and Whitlock3,Reference Ellacott and Cone51) . When in positive energy balance, Small-tailed Han sheep had greater protein expressions of POMC, MC3R, MC4R and CART, which were consistent with their greater mRNA expressions than in Tibetan sheep. This suggested greater energy expenditure in Small-tailed Han than in Tibetan sheep, which supported the greater maintenance energy requirements and lower BW gain in Small-tailed Han than in Tibetan sheep(Reference Jing, Zhou and Wang21). Moreover, AgRP is an endogenous MC3R and MC4R antagonist(Reference Ellacott and Cone51,Reference Morton and Schwartz52) ; consequently, the lesser MC3R and MC4R expressions in Tibetan sheep were consistent with their greater AgRP expression.
The hypothalamic pathways regulate energy homoeostasis by integrating peripheral signals, which provide information both on the immediate energy status of the body and the long-term energy stores. The major sources of these signals are the gastrointestinal tract, the pancreas and the adipose tissue(Reference Roche, Blache and Kay1). The gastrointestinal tract is the largest endocrine organ in the body, and nutrient sensors are positioned strategically in enteroendocrine cells to regulate the secretion of gut peptides, such as ghrelin, GIP and GLP-1, which play vital roles in the acute modulation of energy homoeostasis, as they are sensitive to the content of nutrients in the gastrointestinal tract(Reference Roche, Blache and Kay1,Reference Murphy and Bloom4,Reference Murphy, Dhillo and Bloom8,Reference Mace and Marshall53,Reference Efeyan, Comb and Sabatini54) . Ghrelin is a circulating peptide hormone produced predominantly in the stomach (abomasum in ruminants), has been identified as having orexigenic effects in both monogastric animals and ruminants(Reference Murphy and Bloom4,Reference Wertz-Lutz, Knight and Pritchard25,Reference Nakazato, Murakami and Date55,Reference Kojima and Kangawa56) and is markedly increased during food deprivation(Reference Bagnasco, Kalra and Kalra57). It was reported that infusion of ghrelin activates the hypothalamic NPY/AgRP neurons to secrete neuropeptides of NPY and AgRP(Reference Cowley, Smith and Diano58,Reference Shintani, Ogawa and Ebihara59) and causes an increase in feed intake(Reference Wertz-Lutz, Knight and Pritchard25). Moreover, ghrelin antagonist overrode the mRNA expression of NPY and modified metabolic hormone receptors in the hypothalamus of feed-restricted ewes(Reference Martin, Parker and Furnus60), which demonstrated that ghrelin plays a key role in the hypothalamic regulation of energy homoeostasis by regulating the expression of neuropeptides. Therefore, in the present study, when in negative energy balance, the greater protein expressions of NPY and AgRP were consistent with the greater pre-prandial serum concentration of ghrelin in Tibetan than in Small-tailed Han sheep. However, in Small-tailed Han sheep, the lower blood ghrelin concentration and NPY and AgRP expressions were consistent with the higher leptin concentration than in Tibetan sheep when in negative energy balance, as leptin inhibits the secretion of ghrelin(Reference Kalra, Ueno and Kalra61). Ghrelin acts as an orexigenic agent to stimulate feeding, and increased following food deprivation but decreased following post-feeding(Reference Kalra, Ueno and Kalra61). In the present study, the concentration of active ghrelin was measured pre-feeding and was higher in Tibetan sheep than Small-tailed Han sheep. This suggested that Tibetan sheep had a greater intake than Small-tailed Han sheep when feed was presented, and this might be the result of the long-term adaption of Tibetan sheep to grazing on the harsh QTP. However, this premise needs more research during post-feeding and at more time points, as the secretion of ghrelin is rhythmic(Reference Bagnasco, Kalra and Kalra57).
GIP and GLP-1 are released from the L enteroendocrine cells of the gut after feed ingestion and act as anorexic agents to activate the POMC/CART pathways(Reference Roche, Blache and Kay1,Reference Murphy and Bloom4,Reference Murphy, Dhillo and Bloom8,Reference Stanley, Wynne and McGowan11,Reference NamKoong, Kim and Lee62) . In the present study, when in negative energy balance, Tibetan sheep had greater protein expression of CART, which was consistent with their higher serum concentrations of GIP and GLP-1 and greater protein expressions of GIPR and glucagon-like peptide-1 receptor than Small-tailed Han sheep. However, when in positive energy balance, Small-tailed Han sheep had higher protein expressions of POMC and CART, which was inconsistent with the lower concentrations of GIP and GLP-1 than Tibetan sheep. This may be a result of a higher capacity of GIP and GLP-1 to cross the blood–brain barrier into the hypothalamus, and the POMC/CART pathway is more sensitive to respond to GIP and GLP-1 in Small-tailed Han than in Tibetan sheep when in positive energy balance(Reference Roche, Blache and Kay1). However, this possibility requires further research. In the present study, Tibetan sheep had higher concentrations of both anorexic and orexigenic agents, which were secreted from the gastrointestinal tract, and greater neuropeptides expression of both NPY/AgRP and POMC/CART when in negative energy balance. These differences suggested Tibetan sheep were more responsive than Small-tailed Han sheep in gut peptides signalling the hypothalamic neuropeptides pathway, especially when in negative energy balance. Therefore, Tibetan sheep had a tighter regulation in energy homoeostasis to cope with low energy intake in the harsh QTP. However, these premises require further research, as the secretion of orexigenic agents is reduced post-feeding, while anorexic agents increase when food enters the lumen of the digestive tract, but these agents were only measured in the blood of pre-feeding in the present study.
Long-term energy reserve signals, such as insulin and leptin, also influence energy homoeostasis by regulating the activity of neuronal pathways(Reference Roche, Blache and Kay1,Reference Schwartz, Woods and Porte9,Reference Morton, Cummings and Baskin10,Reference Schwartz, Baskin and Kaiyala63) . Leptin and insulin increase expressions of the anorexigenic neuropeptides POMC and CART but decrease expressions of the orexigenic neuropeptides NPY and AgRP in the hypothalamus(Reference Stanley, Wynne and McGowan11,Reference Sorensen, Adam and Findlay64–Reference Schwartz, Figlewicz and Baskin68) . Adipose tissue is the largest and most effective energy depot in mammals, reflects a long-term energy storage status and plays a vital role in the regulation of energy metabolism and balance(Reference Konige, Wang and Sztalryd69,Reference Rajala and Scherer70) . Leptin and insulin, key signals in the regulation of energy storage and mobilisation in the long-term, are secreted in proportion to body adipose tissue and enter the hypothalamic neuronal system in proportion to their circulation concentrations(Reference Stanley, Wynne and McGowan11,Reference Morton and Schwartz52,Reference Schwartz, Figlewicz and Baskin68,Reference Schwartz, Peskind and Raskind71,Reference Bagdade, Bierman and Porte72) . Adam et al. (Reference Adam, Archer and Findlay73) reported that hypothalamic pathway changes were consistent with responses to blood leptin concentration rather than the leptin receptor expression in the hypothalamus. Therefore, in the present study, the greater POMC and CART expressions in Small-tailed Han sheep were consistent with their greater serum concentrations of leptin and insulin than in Tibetan sheep, as was reported previously in these same sheep(Reference Jing, Zhou and Degen23). However, Tibetan sheep had greater protein expressions of leptin and insulin receptors than Small-tailed Han sheep, which suggests that Tibetan sheep had a greater capacity to transport leptin and insulin across the blood–brain barrier to the hypothalamus than Small-tailed Han sheep.
Additionally, AMPK was identified as playing a vital role in the whole-body energy homoeostasis regulation in the central nervous system by responding to hormones and nutrient signals(Reference Lage, Diéguez and Vidal-Puig13,Reference López, Nogueiras and Tena-Sempere14,Reference Hardie74,Reference Kahn, Alquier and Carling75) . The hypothalamic AMPK activity in the regulation of energy homoeostasis is modulated by both the peripheral and central neuropeptide signals in the hypothalamus. Hypothalamic AMPK activity is inhibited by anorectic signals such as leptin and insulin but is enhanced by orexigenic signals such as ghrelin(Reference Minokoshi, Alquier and Furukawa76–Reference Kubota, Yano and Kubota78). A negative energy balance stimulates AMPK, which increases food intake and weight gain, whereas inhibition of AMPK activity promotes weight loss, at least in rodents(Reference Lage, Diéguez and Vidal-Puig13,Reference Minokoshi, Alquier and Furukawa76,Reference Andersson, Filipsson and Abbott77) . Therefore, the greater AMPKα2 protein expression in Tibetan sheep was consistent with the greater serum concentration of ghrelin and lesser concentrations of leptin and insulin than in Small-tailed Han sheep and was also consistent with the lesser BW loss when in negative energy balance but greater BW gain when in positive energy balance than Small-tailed Han sheep. Besides hormonal signals from the periphery, neuropeptides involved in the regulation of hypothalamic AMPK activity are increased by AgRP but inhibited by POMC(Reference Claret, Smith and Batterham12,Reference Minokoshi, Alquier and Furukawa76) . In addition, AMPK is essential for the regulation of the expressions of orexigenic and anorexigenic neuropeptides(Reference Claret, Smith and Batterham12–Reference Oh, Cho and Cho15). This can explain, at least in part, the greater protein expression of AMPKα2 and AgRP in Tibetan than in Small-tailed Han sheep, and the greater POMC protein expression but lower AMPKα2 protein expression in Small-tailed Han than in Tibetan sheep. Furthermore, these responses demonstrated that the hormonal signals induced changes in hypothalamic AMPK activity, which was consistent with the changes in neuropeptide expressions of NPY/AgRP and POMC/CART in the ARC, as the AMPK provides a link between peripheral signals and central nervous energy homoeostasis regulation pathways. However, the greater AMPKα2 mRNA expression did not lead to a greater AMPKα protein expression in Small-tailed Han than in Tibetan sheep, which suggests that Tibetan sheep had a greater capacity of transcription regulation in AMPKα2 protein expression, as in the NPY/AgRP pathway protein expression.
Conclusions
In hypothalamic regulation of energy homoeostasis, Tibetan sheep displayed greater protein expressions of NPY and AgRP when in negative energy balance, but lesser protein expressions of POMC and CART when in positive energy balance, as well as a greater AMPKα2 protein expression than Small-tailed Han sheep. These responses explained, at least in part, the lesser BW loss when in negative energy balance, the greater BW gain when in positive energy balance and lesser energy requirement for maintenance in Tibetan than in Small-tailed Han sheep. Small-tailed Han sheep were more sensitive to the regulation of anorexigenic gut peptides, GLP-1 and GIP than Tibetan sheep when in positive energy balance; however, Tibetan sheep were more sensitive to the regulation of both anorexic and orexigenic gut peptides when in negative energy balance. These differences conferred Tibetan sheep an advantage over Small-tailed Han sheep in coping with low energy intake on the harsh QTP. These findings provide: (1) an important basis for further studies of hypothalamic regulation of energy homoeostasis and (2) support the relevance of such studies across animal species.
Acknowledgements
The authors are grateful to three anonymous reviewers for their very helpful suggestion on this paper and Deli Liu for animal care, and Chao Yang, Fuyu Shi, Na Guo, Qi Yan, Mingjie Hou, Jiaojiao Zhang, Weixing Xu and Sisi Bi for help in collecting samples.
This work was supported by the National Natural Science Foundation of China (31601960 and 32072757). Xiaoping Jing was supported by the Chinese Scholarship Council (CSC, China).
R. L., J. Z. and X. J. conceived and designed the experiment. X. J., J. Z., W. W., Y. G. and J. K., performed the experiment. X. J., A. D., L. D., Z. S. and R. L. contributed to the writing and revising of the manuscript. All authors read and approved the final manuscript.
The authors declare no competing or financial interests.
Supplementary material
For supplementary materials referred to in this article, please visit https://doi.org/10.1017/S0007114521001902