Introduction
Numerous species worldwide exist in small populations. In some cases this is a natural phenomenon that occurs in populations adapted to scarce or fragmented habitats. The small-population paradigm holds that these populations are at risk of extinction due to rarity or small size itself (Caughley Reference Caughley1994). The effects of inbreeding depression, genetic drift and demographic stochasticity acting alone or together can reduce reproduction and increase mortality resulting in even lower population sizes which in turn leads to more inbreeding and greater effects of genetic drift, a process also known as the extinction vortex (Gilpin and Soulé Reference Gilpin, Soulé and Soulé1986). Examples of naturally occurring small populations include the Lord Howe Woodhen Hypotaenidia sylvestris, restricted to Lord Howe Island; the Straight-billed Reedhaunter Limnoctites rectirostris, a furnariid that occurs in extreme southern Brazil, southern Uruguay and eastern Argentina in small marshes and swales (Ridgely and Tudor Reference Ridgely and Tudor2009) and the South Georgia Pipit Anthus antarcticus, a bird that is endemic to the sub-Antarctic island of South Georgia. Another scenario, called the declining-population paradigm (Caughley Reference Caughley1994), posits that a population is in decline because something external to it has been modified. Low population sizes are often a consequence of anthropogenic influence such as habitat destruction, pollution, and invasive species, among others. Examples of the declining-population paradigm include the ‘Critically Endangered’ Kakapo Strigops habroptila, a parrot known to survive in only three small offshore New Zealand islands (Clout and Merton Reference Clout and Merton1998). There were no land mammals in New Zealand prior to human settlement, except for bats. The combination of flightlessness, solo parentage, nocturnal behaviour, altricial young, and ground-nesting made Kakapo an easy target for mammalian predators which drove the species to the brink of extinction (Lloyd and Powlesland Reference Lloyd and Powlesland1994, Clout and Merton Reference Clout and Merton1998). Another example is the California Condor Gymnogyps californianus, a species that in 1982 had a world population of 22 individuals (Snyder and Snyder Reference Snyder and Snyder2000). The main threats to condors are persecution (shooting and poisoning), unintentional lead poisoning and loss of wildlands (Finkelstein et al. Reference Finkelstein, Doak, George, Burnett, Brandt, Church, Grantham and Smith2012). A third example is the North Island Brown Kiwi Apteryx australis mantelli which only remains scattered in small islands of forest and scrub left after large-scale forest clearance for farmland use on New Zealand’s North Island (Potter Reference Potter1990).
Once populations become smaller and more fragmented, interactions with other species such as predation, mutualism or parasitism can more strongly influence population trajectories and community structure (Bennet and Saunders Reference Bennet, Saunders, Sodhi and Ehrlich2010). A growing number of studies have focused on how a change in species abundance or the loss of a species can impact ecological processes in fragmented habitats. For example, fragmentation of habitats can increase the proportional amount of forest edge, which in turn, can change predator-prey relationships as these edges allow generalist predators access to birds that nest in these fragments (Andrén 1992, Flaspohler et al. Reference Flaspohler, Temple and Rosenfield2001). Other effects of population declines include disruption in seed dispersal of large-seeded plants after reduction of frugivorous bird and bat species in subtropical rainforest fragments (Moran et al. Reference Moran, Catterall and Kanowski2009) and increased aggressive competition in bird communities in landscapes fragmented due to human activity (Maron and Kennedy Reference Maron and Kennedy2007). Each situation is particular to region, taxon and context, which is why the effect of fragmentation cannot be easily generalised (Bennet and Saunders Reference Bennet, Saunders, Sodhi and Ehrlich2010).
Here we focus on the effect of Philornis nest parasitism on Neotropical bird species that are already present at low population sizes. Because parasitism exerts extra pressure on already small or declining populations, we call it an additive negative effect of parasitism, in agreement with Delannoy and Cruz (Reference Delannoy, Cruz, Loye and Zuk1991). While in a large population the effects of nest parasitism may be negligible, in fragmented and small size populations its effects could be devastating. However, field studies focusing on the detrimental effects of parasitism on small and declining bird populations are few and scattered. We review five examples that illustrate this and include a new case not previously discussed in the literature. The six cases fit into the declining-population paradigm since for all cases, population sizes are extremely low. First, we discuss the introduction of Philornis downsi into the Galápagos Islands, emphasizing its effects on Mangrove Finch Camarhynchus heliobates and Medium Tree Finch C. pauper populations. Second, we discuss the case of P. pici parasitizing Ridgway’s Hawk Buteo ridgwayi in the Dominican Republic. Third, we discuss the status of Sharp-shinned Hawk Accipiter striatus vennator populations in Puerto Rico parasitized by P. pici and P. obscura. Fourth, we discuss the Yellow Cardinal Gubernatrix cristata being attacked by an unidentified Philornis species in Argentina. There are other reports of unidentified Philornis species attacking rare or threatened bird species, including the parrot Amazona vitatta in Puerto Rico (Snyder et al. Reference Snyder, Wiley and Kepler1987 cited by Delannoy and Cruz Reference Delannoy, Cruz, Loye and Zuk1991) and the Choco Screech Owl Megascops guatemalae centralis in Ecuador (Reyes and Astudillo-Sánchez Reference Reyes and Astudillo-Sánchez2017) but we focus here on the published cases that present the most information (Table 1). Lastly, we include a newly reported case of Philornis parasitism on the endemic and rare Esmeraldas Woodstar Chaetocercus berlepschi of western Ecuador whose effects on host fledging rates are currently unknown (Figure 1). The goal of this review is to raise awareness of the additional negative effects that nest parasites can bring upon bird populations that are small and declining, by accelerating the population decline.
Table 1. Information on Philornis species with additive mortality effects on species (or subspecies) of Neotropical birds of conservation concern. Common names in bold refer to the (sub)species discussed in the text.
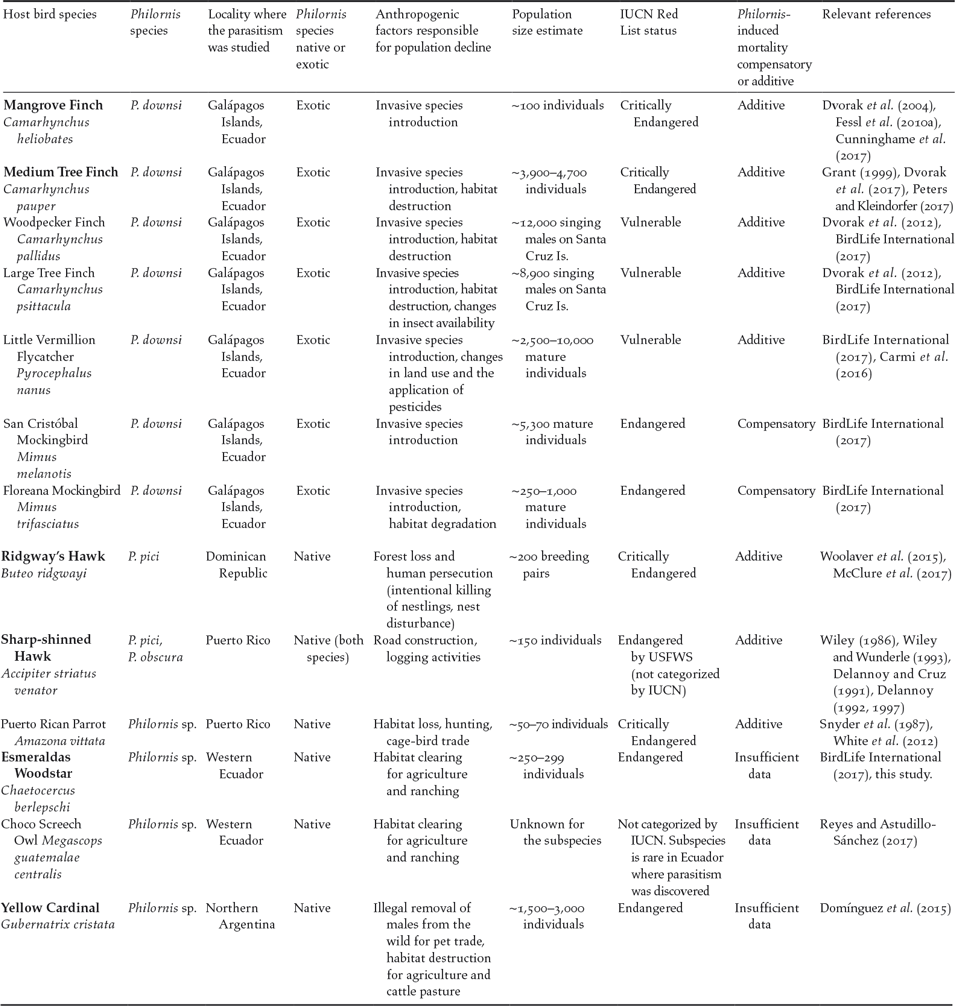

Figure 1. Map showing the six species of small and declining bird populations suffering the additive effects of Philornis parasitism in the Neotropics. Note: Galápagos Islands are not to scale. Photo credits: Mangrove Finch by Francesca Cunninghame, Medium Tree Finch by Sonia Kleindorfer, Esmeraldas Woodstar by Berton Harris, Ridgway’s Hawk by Thomas Hayes, Sharp-shinned Hawk by Julio C. Gallardo, and Yellow Cardinal by Mariana Bulgarella.
Biology of Philornis flies
The genus Philornis is comprised of ∼ 50 species of Neotropical muscid flies (Couri et al. Reference Couri, Carvalho and Löwenberg-Neto2007). Some information on the ecology and life cycle is known for about half of these, all of which are intimately associated with bird nests (Couri Reference Couri, Guimaraes and Papavero1999, Teixeira Reference Teixeira, Guimaraes and Papavero1999, Dudaniec and Kleindorfer Reference Dudaniec and Kleindorfer2006, Fessl et al. Reference Fessl, Sinclair and Kleindorfer2006a, Kleindorfer and Dudaniec Reference Kleindorfer and Dudaniec2016). While adult Philornis are free-living their larvae complete development within bird nests. The larvae of most species are parasites on nestlings, but at least two are coprophagous, feeding on excrement and other material within nests. The larvae of most of the parasitic species feed subcutaneously on nestlings, but in at least two species (P. downsi and P. falsificus) the late-stage larvae feed ectoparasitically on nestlings (Figure 2). Collectively, Philornis species parasitise a broad range of landbird species in the Neotropical region that produce altricial young, but the host range can vary greatly among species (Dodge and Aitken Reference Dodge and Aitken1968, Teixeira Reference Teixeira, Guimaraes and Papavero1999, Dudaniec and Kleindorfer Reference Dudaniec and Kleindorfer2006, Löwenberg-Neto Reference Löwenberg-Neto2008, Bulgarella and Heimpel Reference Bulgarella and Heimpel2015).
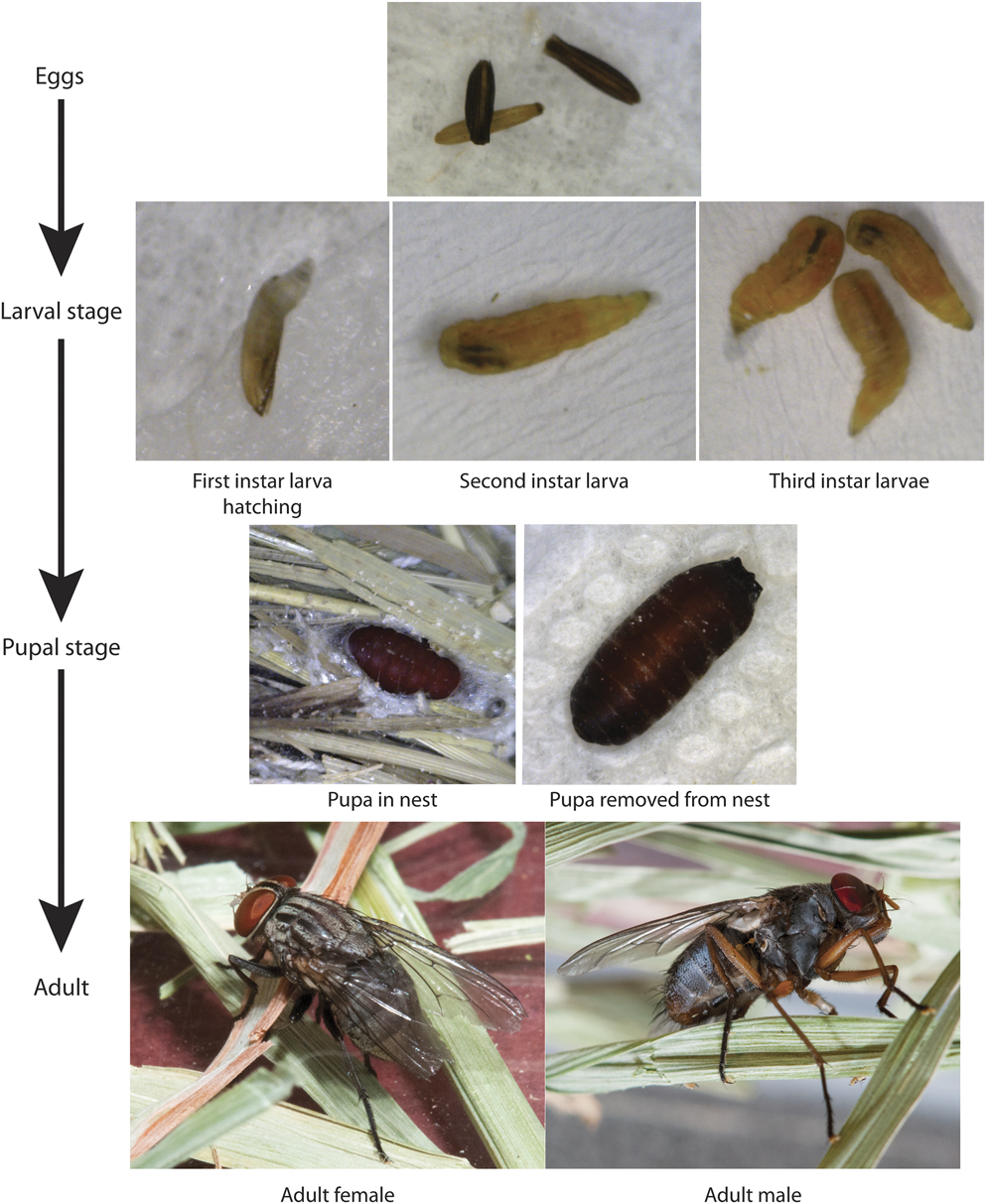
Figure 2. Life cycle of Philornis downsi from egg to adult. Photo credits: Eggs, larvae and pupae photos by Mariana Bulgarella, adult fly photos by David Hansen.
Effects of Philornis on host birds
Philornis parasites can cause substantial levels of nestling mortality in host birds. The fitness effects imposed on bird populations varies greatly depending on which species of Philornis and host are involved (Dudaniec and Kleindorfer Reference Dudaniec and Kleindorfer2006, Kleindorfer and Dudaniec Reference Kleindorfer and Dudaniec2016, Manzoli et al. Reference Manzoli, Saravia-Pietropaolo, Antoniazzi, Barengo, Arce, Quiroga and Beldomenico2018). In addition, a number of ecological, demographic and behavioural factors determine the effects of Philornis parasitism on bird fitness. These include the per-nestling level of infestation (Arendt Reference Arendt1985, Fessl and Tebbich Reference Fessl and Tebbich2002, Fessl et al. Reference Fessl, Kleindorfer and Tebbich2006b, Koop et al. Reference Koop, Huber, Laverty and Clayton2011, Knutie et al. Reference Knutie, Owen, McNew, Bartlow, Arriero, Herman, DiBlasi, Thompson, Koop and Clayton2016, Heimpel et al. Reference Heimpel, Hillstrom, Freund, Knutie and Clayton2017), the timing of nestling infestation (Arendt Reference Arendt2000, Kleindorfer et al. Reference Kleindorfer, Peters, Custance, Dudaniec and O’Connor2014a), parental provisioning in relation to parasite-weakened begging (O’Connor et al. Reference O’Connor, Robertson and Kleindorfer2014), sibling competition, Philornis spp. consumption by the birds (O’Connor et al. Reference O’Connor, Robertson and Kleindorfer2010a), and the availability of food for nestlings, which is itself often determined by environmental conditions (Arendt Reference Arendt2000, Dudaniec et al. Reference Dudaniec, Fessl and Kleindorfer2007, Antoniazzi et al. Reference Antoniazzi, Manzoli, Rohrmann, Saravia, Silvestri and Beldomenico2011, Koop et al. Reference Koop, Le Bohec and Clayton2013a, Manzoli et al. Reference Manzoli, Antoniazzi, Saravia, Silvestri, Rorhmann and Beldomenico2013, Cimadom et al. Reference Cimadom, Ulloa, Meidl, Zöttl, Zöttl, Fessl, Nemeth, Dvorak, Cunninghame and Tebbich2014).
Much of the information on Philornis effects on bird fitness has been gained from experimental field studies on P. downsi in the Galápagos Islands, where it is an invasive parasite of landbirds including Darwin’s Finches (Kleindorfer and Dudaniec Reference Kleindorfer and Dudaniec2016, Fessl et al. Reference Fessl, Heimpel, Causton and Parker2018, McNew and Clayton Reference McNew and Clayton2018; see below). Larval feeding by P. downsi on Darwin’s Finches can cause anaemia, beak scarring and death of infested nestlings (Dudaniec et al. Reference Dudaniec, Kleindorfer and Fessl2006, Galligan and Kleindorfer Reference Galligan and Kleindorfer2009, Fessl et al. Reference Fessl, Kleindorfer and Tebbich2006b, Huber et al. Reference Huber, Owen, Koop, King, Grant, Grant and Clayton2010, Kleindorfer and Sulloway Reference Kleindorfer and Sulloway2016). Mean nestling mortality is estimated at 55%, but it varies from 3% to 100% (reviewed by Kleindorfer and Dudaniec Reference Kleindorfer and Dudaniec2016). Population viability modelling has suggested that local or global extinction as a result of P. downsi infestation is a distinct possibility for some species of Darwin’s Finches (Fessl et al. Reference Fessl, Young, Young, Rodríguez-Matamoros, Dvorak and Tebbich2010a, Koop et al. Reference Koop, Kim, Knutie, Adler and Clayton2016; see below).
Host responses to Philornis
Little is known about behavioural or physiological defences mounted by bird hosts to Philornis parasitism. Removal of Philornis larvae by adult birds (a behavioural defence) has been observed in Galápagos (O’Connor et al. Reference O’Connor, Robertson and Kleindorfer2010a). However, in most Philornis-host associations, this behaviour is considered not to occur at meaningful levels (Fraga Reference Fraga1984, Koop et al. Reference Koop, Owen, Knutie, Aguilar and Clayton2013b, Fessl et al. Reference Fessl, Heimpel, Causton and Parker2018). Also in Galápagos, adult birds have been observed probing the base of the nest to remove larvae and nestlings compete to stand on top of each other in order to avoid larvae on the nest base, as recorded on video: https://www.youtube.com/watch?v=YfkMFxBZSns (Kleindorfer and Dudaniec Reference Kleindorfer and Dudaniec2016). Another potential behavioural defence involves the use of repellent substances by host birds. Certain birds place green materials in their nests; these are not part of the nest structure, but they are placed on the inside or the edges of the nest (Wimberger Reference Wimberger1984). The nest protection hypothesis states that green plants decrease nest parasites or pathogens through their secondary compounds, benefiting the nestlings (Wimberger Reference Wimberger1984). Cimadom et al. (Reference Cimadom, Causton, Cha, Damiens, Fessl, Hood-Nowotny, Lincango, Mieles, Nemeth, Semler, Teale and Tebbich2016) have recently discovered that some species of Darwin’s Finches rub their feathers with leaves of an endemic Galápagos plant that has repellent properties to P. downsi and mosquitoes. The extent to which this activity protects these birds from Philornis infection is not known. In terms of physiological defences, brooding females of Darwin’s Finches have been shown to produce antibodies in response to P. downsi infection in some studies (Huber et al. Reference Huber, Owen, Koop, King, Grant, Grant and Clayton2010, Koop et al. Reference Koop, Owen, Knutie, Aguilar and Clayton2013b) but not others (Knutie et al. Reference Knutie, Owen, McNew, Bartlow, Arriero, Herman, DiBlasi, Thompson, Koop and Clayton2016, Reference Knutie, Herman, Owen and Clayton2017). However, even in cases where an immune response was detected, this response was not effective at helping nestlings survive parasitism (Koop et al. Reference Koop, Owen, Knutie, Aguilar and Clayton2013b).
Other bird species, however, seem to tolerate Philornis parasitism more easily. Several mockingbird species are attacked by various Philornis species (Fraga Reference Fraga1984, Löwenberg-Neto Reference Löwenberg-Neto2008, Bulgarella and Heimpel Reference Bulgarella and Heimpel2015). A recent study showed that Mimus parvulus and M. gilvus exhibited high levels of tolerance to P. downsi and P. trinitensis, respectively (Knutie et al. Reference Knutie, Owen, McNew, Bartlow, Arriero, Herman, DiBlasi, Thompson, Koop and Clayton2016, Reference Knutie, Herman, Owen and Clayton2017). Nestlings of these species can tolerate heavy Philornis parasitism without suffering decreased fledging rates or weights although parasitism by P. trinitensis did reduce the length of the primary feather and tarsus of M. gilvus fledglings (Knutie et al. Reference Knutie, Herman, Owen and Clayton2017). In a study of the Galápagos Mockingbird M. parvulus, nestlings in parasitised nests exhibited enhanced begging behaviour and this resulted in increased parental feeding, which can likely compensate for blood lost to parasitism (Knutie et al. Reference Knutie, Owen, McNew, Bartlow, Arriero, Herman, DiBlasi, Thompson, Koop and Clayton2016). Another study in central Argentina compared the resistance and tolerance of three host bird species that have co-evolved with Philornis torquans under natural conditions. Great Kiskadees Pitangus sulphuratus showed no detectable effect of larval infestation on survival, exhibiting tolerance rather than resistance to parasitism. On the other hand, two species of thornbirds (Phacellodomus ruber and P. sibilatrix) mounted inflammatory responses that demonstrate investment in a resistance response to P. torquans parasitism (Manzoli et al. Reference Manzoli, Saravia-Pietropaolo, Antoniazzi, Barengo, Arce, Quiroga and Beldomenico2018). Of the two thornbird species, P. sibilatrix exhibited less tolerance and presented lower parasite loads leading to lower effects of Philornis on survival, growth and mean virulence in this species than on P. ruber, which presented much higher numbers of larvae (Manzoli et al. Reference Manzoli, Saravia-Pietropaolo, Antoniazzi, Barengo, Arce, Quiroga and Beldomenico2018). Despite this difference, virulence was relatively high for both thornbird species, leading to substantial levels of host mortality. This study showed how a tolerant Philornis host (the Great Kiskadee) can serve as a ‘reservoir host’ that puts less tolerant hosts (thornbirds) at increased risk of attack by producing high numbers of parasites. A similar argument has been made for P. downsi and P. trinitensis attacking both larger-bodied tolerant host species and smaller host species that suffer more negative fitness effects of parasitism (Knutie et al. Reference Knutie, Owen, McNew, Bartlow, Arriero, Herman, DiBlasi, Thompson, Koop and Clayton2016, Reference Knutie, Herman, Owen and Clayton2017; Heimpel et al. Reference Heimpel, Hillstrom, Freund, Knutie and Clayton2017).
Philornis downsi in the Galápagos Islands
As noted above, P. downsi is invasive in the Galápagos Islands where it was introduced from its native range in mainland South America sometime before or during the 1960s (Causton et al. Reference Causton, Peck, Sinclair, Roque-Albelo, Hodgson and Landry2006, Bulgarella et al. Reference Bulgarella, Quiroga, Brito Vera, Dregni, Cunninghame, Mosquera Muñoz, Monje, Causton and Heimpel2015, Kleindorfer and Sulloway Reference Kleindorfer and Sulloway2016, Fessl et al. Reference Fessl, Heimpel, Causton and Parker2018). This parasite is having a stronger effect on various species of Darwin’s Finches in Galápagos than it does on birds in its native range, possibly because it has escaped enemies such as parasitoids and possibly ants (Bulgarella et al. Reference Bulgarella, Quiroga, Brito Vera, Dregni, Cunninghame, Mosquera Muñoz, Monje, Causton and Heimpel2015, Reference Bulgarella, Quiroga, Boulton, Ramírez, Moon, Causton and Heimpel2017, Delvare et al. Reference Delvare, Heimpel, Baur, Chadee, Martinez and Knutie2017, Knutie et al. Reference Knutie, Herman, Owen and Clayton2017). However, using in-nest videos in Galápagos, researchers found one instance in which small ants removed P. downsi larvae from a Darwin’s Finch nest during the daytime (O’Connor et al. Reference O’Connor, Robertson and Kleindorfer2010a). Of the 17 recognized species of Darwin’s Finches in Galápagos, 11 have been documented as hosts for P. downsi (Fessl et al. Reference Fessl, Heimpel, Causton and Parker2018). Of these, two – the Mangrove Finch and the Medium Tree Finch – are ‘Critically Endangered’ and P. downsi is implicated in their declines as we discuss below. Philornis downsi has also been suggested as a possible contributing factor for island-level extinctions or near-extinctions of the Grey Warbler Finch Certhidea fusca and Vegetarian Finch Platyspiza crassirostris and the Vermilion Flycatcher Pyrocephalus nanus on Floreana Island (Grant et al. Reference Grant, Grant, Petren and Keller2005, Dvorak et al. Reference Dvorak, Nemeth, Wendelin, Herrera, Mosquera, Anchundia, Sevilla, Tebbich and Fessl2017, Peters and Kleindorfer Reference Peters and Kleindorfer2017) and is implicated in a steep decline of the Green Warbler Finch Certhidea olivacea population in the highlands of Santa Cruz Island (Cimadom et al. Reference Cimadom, Ulloa, Meidl, Zöttl, Zöttl, Fessl, Nemeth, Dvorak, Cunninghame and Tebbich2014). In addition, population viability analyses suggest that populations of the abundant Medium Ground Finch Geospiza fortis may go extinct within the next 50–100 years depending upon ecological conditions (Koop et al. Reference Koop, Kim, Knutie, Adler and Clayton2016). It is also worth noting that recent genetic analyses of Vermillion Flycatchers have shown that the San Cristóbal endemic Pyrocephalus dubius went extinct on the island of San Cristóbal some time before the 1980s (Carmi et al. Reference Carmi, Witt, Jaramillo and Dumbacher2016). Philornis downsi is known to attack a sister species, the Vermilion Flycatcher P. nanus classified as ‘Vulnerable’ on the IUCN Red List (Fessl and Tebbich Reference Fessl and Tebbich2002) and the fly is present on San Cristóbal Island (Wiedenfeld et al. Reference Wiedenfeld, Jiménez, Fessl, Kleindorfer and Valarezo2007) making it conceivable that P. downsi played a role in the extinction of the San Cristóbal Vermillion Flycatcher.
The Mangrove Finch and the Medium Tree Finch in the Galápagos Islands
The Mangrove Finch Camarhynchus heliobates is one of the rarest bird species in the world, with an estimated population size of approximately 80 to 100 individuals (Dvorak et al. Reference Dvorak, Vargas, Fessl and Tebbich2004, Fessl et al. Reference Fessl, Young, Young, Rodríguez-Matamoros, Dvorak and Tebbich2010a, Cunninghame et al. Reference Cunninghame, Fessl, Sevilla, Young and La Greco2017). It is classified as ‘Critically Endangered’ on the IUCN Red List of Threatened Species (henceforth IUCN Red List) (BirdLife International 2017). The Mangrove Finch is a mangrove habitat forest specialist (Young et al. Reference Young, Cunninghame, Fessl, Vargas, Gleason and Victor2013) with a historically small population, fitting in the small-population paradigm. The species’ distribution used to include mangrove forests on the islands of Fernandina and Isabela, however, it disappeared from Fernandina sometime in the 1990s (Grant and Grant Reference Grant and Grant1997). The remaining Mangrove Finch population is currently restricted to two coastal mangrove forests on north-western Isabela Island.
Both human-induced and natural causes have led to the decline of the Mangrove Finch. Among the human-induced factors, invasive species have been perhaps the most important, and this includes effects of ship rats Rattus rattus that are predatory upon the eggs and nestlings, the yellow paper wasp Polistes versicolor that competes for food (Grant and Grant Reference Grant and Grant1997), and critically, the fly Philornis downsi that produces high nestling mortality (Fessl et al. Reference Fessl, Young, Young, Rodríguez-Matamoros, Dvorak and Tebbich2010a, Lawson et al. Reference Lawson, Fessl, Vargas, Farrington, Cunninghame, Mueller, Nemeth, Sevilla and Petren2017). Grant and Grant (Reference Grant and Grant1997) also noted that cutting mangroves may have been particularly detrimental, especially in the Villamil area of southern Isabela, which is now devoid of Mangrove Finches. Natural causes include habitat alteration due to volcanic uplift and hybridisation with the closely related Woodpecker Finch C. pallidus, classified as ‘Vulnerable’ on the IUCN Red List (Lawson et al. Reference Lawson, Fessl, Vargas, Farrington, Cunninghame, Mueller, Nemeth, Sevilla and Petren2017). These and other causes have all contributed to pushing this species to the brink of extinction (Lawson et al. Reference Lawson, Fessl, Vargas, Farrington, Cunninghame, Mueller, Nemeth, Sevilla and Petren2017).
A recovery plan for the Mangrove Finch was developed in 2010 (Fessl et al. Reference Fessl, Vargas, Carrión, Young, Deem, Rodríguez-Matamoros, Atkinson, Carvajal, Cruz, Tebbich and Young2010b). Conservation management since has included successful rat control within the Mangrove Finch range and a trial translocation, where nine birds were relocated to an area previously occupied by the species in May 2010, in an attempt to increase its geographic range. Unfortunately, four of the birds returned to the source population and none have been sighted in the translocation area since November 2010 (Cunninghame et al. Reference Cunninghame, Young, Sevilla, Carrión and Fessl2013). By 2011, with successful rat control adopted as a management technique, parasitism by P. downsi was identified as the main factor responsible for causing nest failures. A more intensive conservation approach, head-starting, started in 2014 aimed at keeping the population buoyant and viable in the short term (Cunninghame et al. Reference Cunninghame, Switzer, Parks, Young, Carrión, Medranda and Sevilla2015). In brief, this technique consists of collecting eggs and/or nestlings from wild nests, artificially incubating eggs and hand-rearing chicks in captivity followed by the release of the juvenile birds back into the wild (Cristinacce et al. Reference Cristinacce, Ladkoo, Switzer, Jordan, Vencatasamy, de Ravel Koenig, Jones and Bell2008, Cunninghame et al. Reference Cunninghame, Switzer, Parks, Young, Carrión, Medranda and Sevilla2015, Fessl et al. Reference Fessl, Heimpel, Causton and Parker2018), effectively circumventing the critical nesting period when P. downsi larvae feed on nestlings. Over four seasons of head-starting, from 2014 to 2017, a total of 39 juvenile Mangrove Finches have been released back into the wild. Captive-reared individuals have been observed surviving into the following breeding season and breeding with wild-reared Mangrove Finches, which demonstrates the success of the technique (F. Cunninghame pers. comm.). However due to the remote location of the remaining Mangrove Finch population, head starting is expensive and logistically challenging, thus a long term and financially sustainable solution to control P. downsi in the Galápagos archipelago is urgently needed.
Another of Darwin’s Finches in steep decline is the Medium Tree Finch Camarhynchus pauper also classified as ‘Critically Endangered’ and restricted to fragmented forest patches in the humid highlands of Floreana Island (Lack Reference Lack1947, Grant Reference Grant1999, Dvorak et al. Reference Dvorak, Nemeth, Wendelin, Herrera, Mosquera, Anchundia, Sevilla, Tebbich and Fessl2017, Peters and Kleindorfer Reference Peters and Kleindorfer2017). Its estimated population size is approximately 3,900–4,700 individuals (Fessl et al. Reference Fessl, Heimpel, Causton and Parker2018). The Floreana Island Camarhynchus species have been studied almost annually since 2004, representing a long-term field study carried out in the Cerro Pajas region of Floreana from 2004 to 2016. Since 2004, 561 active nests have been monitored, of which 196 belong to Medium Tree Finches (Kleindorfer et al. Reference Kleindorfer, Peters, Custance, Dudaniec and O’Connor2014a). Therefore, an excellent record for this species is available. Camarhynchus pauper declined by 52% between 2004 and 2013. In 2013, an estimated total of ∼ 419 males remained in the Scalesia forest habitat and ∼ 2,537 males in the entire highland habitat of Floreana Island (Peters and Kleindorfer Reference Peters and Kleindorfer2017). The main concerns for this species include habitat degradation, predation and Philornis parasitism (O’Connor et al. Reference O’Connor, Sulloway, Robertson and Kleindorfer2010b). Floreana Island has the longest history of human habitation within the archipelago. Consequently, extensive clearance of the highland areas for agriculture left only fragmented and invaded habitats for mid-to-high elevation birds (O’Connor et al. 2010c). In addition, these habitats experienced invasion by introduced plants and predators and high levels of nest parasitism (O’Connor et al. Reference O’Connor, Sulloway, Robertson and Kleindorfer2010b). In one season, P. downsi parasitism was responsible for 41% mortality of Medium Tree Finch nestlings (O’Connor et al. Reference O’Connor, Sulloway, Robertson and Kleindorfer2010b). This species presents the highest number of P. downsi per nest of any bird species on Floreana Island. Medium Tree Finches presented significantly higher parasite intensity (54.7 ± 5.4) when compared with the more common species, Small Tree Finch C. parvulus (28.7 ± 2.4) and Small Ground Finch Geospiza fuliginosa (31.0 ± 2.1) (Kleindorfer et al. Reference Kleindorfer, Peters, Custance, Dudaniec and O’Connor2014a). A 10-year study carried out from 2004 to 2013, demonstrated a decrease in host age at death from ∼ 11 to ∼ 5 days old, an increase in parasite intensity from ∼ 28 to ∼ 48 parasites per nest, and an increase in host mortality from ∼ 50% to ∼ 90% (Kleindorfer et al. Reference Kleindorfer, Peters, Custance, Dudaniec and O’Connor2014a). Interestingly, nesting height was shown to predict P. downsi intensity in tree finch species on Floreana Island (Kleindorfer et al. Reference Kleindorfer, Peters, Hohl, Sulloway, Weiss and Sol2016). The Medium Tree Finch presents the highest nesting height at the approximate altitude where traps catch the largest number of P. downsi flies, which suggests that the high number of parasites found on this species might be related to the parasite flight behaviour rather than specific host attributes per se (Kleindorfer et al. Reference Kleindorfer, Peters, Hohl, Sulloway, Weiss and Sol2016).
Furthermore, Medium Tree Finch females preferentially pair with Small Tree Finch males, driving asymmetrical introgression (Kleindorfer et al. Reference Kleindorfer, O’Connor, Dudaniec, Myers, Robertson and Sulloway2014b, Peters et al. Reference Peters, Myers, Dudaniec, O’Connor and Kleindorfer2017). Interestingly, these hybrids had fewer P. downsi parasites per nest than pure Medium Tree Finch (Kleindorfer et al. Reference Kleindorfer, O’Connor, Dudaniec, Myers, Robertson and Sulloway2014b). These studies suggest that hybridisation may be favoured by natural selection if the hybrids present higher reproductive success due to lower parasitism. As a result, the Medium Tree Finch as a species could disappear through reproductive absorption (Kleindorfer et al. Reference Kleindorfer, O’Connor, Dudaniec, Myers, Robertson and Sulloway2014b, Peters et al. Reference Peters, Myers, Dudaniec, O’Connor and Kleindorfer2017).
A recent study reported significant differences in the microbiome of P. downsi sampled from several host birds from the Galápagos Islands (Ben-Yosef et al. Reference Ben-Yosef, Zaada, Dudaniec, Pasternak, Jurkevitch, Smith, Causton, Lincango, Tobe, Mitchell, Kleindorfer and Yuval2017). The P. downsi microbiome differed between the life stages (larval vs. adults) and according to the feeding guild of the host bird species. The microbiome of the insectivorous Green Warbler Finch Certhidea olivacea was significantly different from the microbiome of other Darwin’s finch species (including the Medium Tree Finch) whose diet presents varying levels of omnivory. It seems that currently, Medium Tree Finches are exposed to the same P. downsi microbiome as other sympatric host species. These findings could inform effective control strategies for this parasite and have implications for understanding novel evolutionary pressures on small host populations (Ben-Yosef et al. Reference Ben-Yosef, Zaada, Dudaniec, Pasternak, Jurkevitch, Smith, Causton, Lincango, Tobe, Mitchell, Kleindorfer and Yuval2017).
Ridgway’s Hawk in the Dominican Republic
Ridgway’s Hawk Buteo ridgwayi is an endemic species that historically occurred only in Haiti and the Dominican Republic (Wiley and Wiley Reference Wiley and Wiley1981, BirdLife International 2000). This hawk is presently extinct in Haiti (Keith et al. Reference Keith, Wiley, Latta and Ottenwalder2003). With an estimated population of ∼ 200 breeding pairs (McClure et al. Reference McClure, Rolek, Hayes, Hayes, Thorstrom, Curti and Anderson2017), it is classified as ‘Critically Endangered’ (BirdLife International 2017). Historically, Ridgway’s Hawk inhabited a wide variety of habitats, being more common in mature secondary forest and small agricultural plots at elevations from sea level to 1,800 m (Wiley and Wiley Reference Wiley and Wiley1981, Thorstrom et al. Reference Thorstrom, Almonte, Balbuena de la Rosa, Bildstein, Barber and Zimmerman2007, Woolaver et al. Reference Woolaver, Nichols, Morton and Stutchbury2013). More than 90% of the original forest cover present in the Dominican Republic has been destroyed by the practice of slash-and-burn agriculture (Harcourt and Ottenwalder Reference Harcourt, Ottenwalder, Harcourt and Sayer1996). The combined effects of forest loss and human persecution including activities such as intentional killing of adults and nestlings as well as the disturbance of nests have restricted the hawk’s distributional range to Los Haitises National Park and surrounding areas in the north-eastern Dominican Republic leading to inbreeding and a recent population bottleneck (Woolaver et al. Reference Woolaver, Nichols, Morton and Stutchbury2013, Reference Woolaver, Nichols, Morton and Stutchbury2015). A five-year-study (2005–2009) conducted at Los Haitises National Park monitored the breeding biology of this hawk species and reported human disturbance as the main cause of nest failure (43%), with parasitism by the native P. pici mentioned among other causes of nest failure (Woolaver et al. Reference Woolaver, Nichols, Morton and Stutchbury2015).
Philornis pici was first described from Santo Domingo in the Dominican Republic by Macquart (Reference Macquart1854). Its larvae feed subcutaneously and until recently little was known about its effect on bird fitness. It parasitises bird host species in the orders Passeriformes, Columbiformes, Piciformes and Psittaciformes (Teixeira Reference Teixeira, Guimaraes and Papavero1999). A study reported that P. pici parasitism reduced the fledging success of this hawk by 179% over one breeding season (Hayes et al. in press). Further monitoring and potential management options for this parasite are desperately needed. A recent translocation of 104 juvenile birds from Los Haitises to Punta Cana has been successful in increasing the geographic range of Ridgway’s Hawk, with hopes this programme will increase overall population sizes (McClure et al. Reference McClure, Rolek, Hayes, Hayes, Thorstrom, Curti and Anderson2017). A Philornis-contingent recovery plan should be put in place to ensure the continued survival of Ridgway’s Hawk as a species.
Sharp-shinned Hawk in Puerto Rico
The Puerto Rican Sharp-shinned Hawk Accipiter striatus vennator is an endemic subspecies of the North American Sharp-shinned Hawk, occurring only in Puerto Rico. It is a small hawk mainly restricted to mature and secondary forests in a few isolated areas of the main island of Puerto Rico. This subspecies has suffered a 40% population decline between 1985 and 1991 with an estimated population size of approximately 150 birds as of 1997 (Delannoy and Cruz Reference Delannoy, Cruz, Loye and Zuk1991, Delannoy Reference Delannoy1992, Bildstein and Meyer Reference Bildstein, Meyer and Poole2000, Ferguson-Lees and Christie Reference Ferguson-Lees and Christie2001), leading to its classification as ‘Endangered’ by the U.S. Fish and Wildlife Service (USFWS 1997). Several factors have contributed to its rapid decline, including road construction, logging activities, predation of eggs and nestlings by the Pearly-eyed Thrasher Margarops fuscatus and parasitism by the Puerto Rican native parasites P. pici and P. obscura (Wiley Reference Wiley1986, Wiley and Wunderle Reference Wiley and Wunderle1993, Delannoy Reference Delannoy1997).
A long-term study carried out at the Maricao Commonwealth Forest (from 1979 to 1983 and in 1985) determined prevalence, parasite load and impact of Philornis parasitism on Sharp-shinned Hawk nestling survival (Delannoy and Cruz Reference Delannoy, Cruz, Loye and Zuk1991). Thirty nests were investigated over the study, 20 of which contained at least one Philornis-infested nestling. Just over half of the 75 nestlings in the study were infested and the average parasite load per nestling was 10 larvae. Fledgling mortality was nearly four times higher for parasitised versus non-parasitised nestlings (61% vs. 18%, respectively) resulting in a significant effect of parasitism on fledging success. Delannoy and Cruz (Reference Delannoy, Cruz, Loye and Zuk1991) concluded that parasitism by Philornis constitutes an additive mortality source for the Puerto Rican Sharp-shinned Hawk and therefore it contributes to population declines of this subspecies. In fact, intensive population censuses conducted at the Maricao Commonwealth Forest between 2012 and 2014 reported no more than seven hawk individuals, suggesting that the Puerto Rican Sharp-shinned Hawk has almost completely disappeared from its known distributional range (Gallardo and Villela 2014). However, no new information on the Philornis-Sharp-shinned Hawk system is available since the studies in the 1980s and 1990s (Gallardo and Villela 2014). It is imperative to produce updated information on the effects of Philornis parasitism and establish a Philornis-specific management plan, if needed, to support successful population recovery.
Yellow Cardinal in Argentina
The Yellow Cardinal Gubernatrix cristata is a passerine endemic to southern South America. This species was historically found throughout Uruguay, southern Brazil and the espinal region (thorny deciduous shrubland forests) of central Argentina (Ridgely and Tudor Reference Ridgely and Tudor2009, Domínguez et al. Reference Domínguez, Reboreda and Mahler2015). However, for over a century, extensive poaching of male Yellow Cardinals for the illegal pet trade (Pessino and Tittarelli Reference Pessino and Tittarelli2006) combined with the destruction of its habitat for agriculture and cattle pasture (Domínguez et al. Reference Domínguez, Reboreda and Mahler2016) have severely affected this species. Yellow Cardinals are now very rare in Brazil; fewer than 300 individuals are believed to remain in Uruguay and the populations in Argentina are discontinuous and reduced in size (Domínguez et al. Reference Domínguez, Reboreda and Mahler2015). Thus, this species is currently classified as ‘Endangered’ on the IUCN Red List (BirdLife International 2017) with an estimated population size of about 1,500–3,000 individuals. In addition, the Yellow Cardinal is also subjected to brood parasitism by the Shiny Cowbird Molothrus bonariensis (Domínguez et al. Reference Domínguez, Reboreda and Mahler2015) and hybridisation with its sister species, the Common Diuca Finch Diuca diuca in Argentina (Bertonatti and López Guerra Reference Bertonatti and López Guerra1997). Furthermore, in a study carried out during the 2011 and 2012 breeding seasons in the northern Argentinian province of Corrientes, four out of 18 nests (22%) examined presented parasitism by an unidentified subcutaneous species of Philornis. Of the four nests, only two fledged nestlings successfully (Domínguez et al. Reference Domínguez, Reboreda and Mahler2015). This study was the first to report Philornis parasitism of Yellow Cardinals. We recommend examining Yellow Cardinal nests throughout its distributional range to determine whether Philornis parasitism is ubiquitous or only occurs in the Argentinian province of Corrientes.
Esmeraldas Woodstar in Ecuador
The Esmeraldas Woodstar Chaetocercus berlepschi is a rare hummingbird species endemic to lowland and foothill garúa forest in western Ecuador (Harris et al. Reference Harris, Agreda, Juiña and Freymann2009). Its distribution is small, restricted, and severely fragmented. The lowland humid forest habitat in western Ecuador is fast disappearing through clearing for agriculture and ranching (Becker and López Lanús Reference Becker and López Lanús1997). For these reasons, the Esmeraldas Woodstar has been classified as ‘Endangered’ on the IUCN Red List. Population size is estimated to be ∼ 250–299 individuals (BirdLife International 2017). Recently, we presented the first record of Philornis parasitism on the Esmeraldas Woodstar (Bulgarella et al. Reference Bulgarella, Quiroga, Boulton, Ramírez, Moon, Causton and Heimpel2017).
During our studies in mainland Ecuador, we sampled previously used wild bird nests once the breeding season finished at the Reserva Ecológica Loma Alta (1.85694°S, 80.59938°W), 17 km inland from the Pacific Ocean in Santa Elena province. A field technician for a different research project monitored a wild Esmeraldas Woodstar nest during the 2015 breeding season. After the fledglings left, the nest was collected on 24 May 2015. When disassembled and inspected, a total of six fly puparia were found; no dead nestlings were found in the nest. Five of these puparia had produced adult flies that emerged prior to nest collection and the other puparium had an unemerged, unidentified parasitoid species inside. Photographs of the empty puparia were sent to Dr Bradley J. Sinclair who confirmed that, based on the spiracular plates, these puparia belonged to a Philornis species (Figure 3). Because we do not have the adult fly specimens we are not able to determine the Philornis species, as the only taxonomic key is based on adult morphology (Couri Reference Couri, Guimaraes and Papavero1999). Although nothing is known of the effects and/or prevalence of Philornis parasitism on the Esmeraldas Woodstar, we strongly recommend further field studies that follow nests of this unique species to make sure that nest parasitism is not interfering with successful breeding and intended population recovery.

Figure 3. Photographs of puparia of an unidentified Philornis species found in an Esmeraldas Woodstar nest on mainland Ecuador. The left panel shows the lateral view of the puparium and the right panel the posterior end of the pupa with the anal spiracles. Photo credits: Mariana Bulgarella.
Discussion
Additive versus compensatory mortality effects on hosts
Bird population sizes are limited by available habitat and food, and by the prevalence of natural enemies including predators, parasites and diseases (Gill Reference Gill2007). The effects of dipteran nest parasites, including Philornis, have long been neglected in avian conservation efforts (Loye and Carroll Reference Loye and Carroll1995, Reference Loye and Carroll1998, Williams et al. Reference Williams, Pople, Showler, Dicks, Child, zu Ermgassen and Sutherland2012). To determine the effects of a parasite on a host population, it is important to know whether the parasite-induced mortality is additive or compensatory. This distinction matters because they have different consequences for the host populations. With compensatory mortality, the host population is not reduced by parasitism, whereas with additive mortality, the host population is reduced at a lower level in the presence of the parasite than in the absence of parasitism (Combes Reference Combes2001). Some of the examples reviewed here are illustrative of the additive effects of Philornis parasitism on small and declining Neotropical bird populations, i.e. the mortality induced by Philornis reduces the bird population size below parasite-free levels. Of the six bird species or subspecies discussed in this review as case studies, additive rather than compensatory effects has been demonstrated for four species (Mangrove Finch, Medium Tree Finch, Ridgway’s Hawk, and Puerto Rican Sharp-shinned Hawk). The data available are not sufficient to distinguish between additive and compensatory mortality due to Philornis parasitism for the remaining two species (Yellow Cardinal and Esmeraldas Woodstar).
Direct and indirect effects of Philornis parasitism
The effects of Philornis parasitism are not restricted to reduced nestling growth and survival (Antoniazzi et al. Reference Antoniazzi, Manzoli, Rohrmann, Saravia, Silvestri and Beldomenico2011, Quiroga and Reboreda Reference Quiroga and Reboreda2012, Rabufetti and Reboreda Reference Rabuffetti and Reboreda2007; see Kleindorfer and Dudaniec Reference Kleindorfer and Dudaniec2016 for a summary of mortality rates in the Galápagos Islands). Birds that survive and fledge from a nest infested by Philornis are still affected by parasitism. Detrimental consequences during the nestling phase include reduced haemoglobin and haematocrit levels (Dudaniec et al. Reference Dudaniec, Kleindorfer and Fessl2006, Fessl et al. Reference Fessl, Kleindorfer and Tebbich2006b), reduced red blood cell counts and boosted white blood cell counts in infected nestlings when compared to nestlings whose larvae were experimentally removed (Manzoli et al. Reference Manzoli, Saravia-Pietropaolo, Antoniazzi, Barengo, Arce, Quiroga and Beldomenico2018), lower body mass (Fessl et al. Reference Fessl, Kleindorfer and Tebbich2006b) and reduced feather and tarsus length (Koop et al. Reference Koop, Huber, Laverty and Clayton2011). Therefore, infested nestlings might be less able to compete with siblings and beg for food which might contribute to early mortality and lower reproductive success (Koop et al. Reference Koop, Huber, Laverty and Clayton2011).
No studies on the long-term effects of Philornis on nestlings that survive parasitism have been conducted for Philornis species with subcutaneous larvae. Studies of longer-term consequences on surviving birds are rare and mostly specific to the Galápagos birds-P. downsi system. As explained above, P. downsi is an ectoparasite but the first instar larvae feed inside the nestling nares. This feeding causes changes in beak structure such as enlarged nares and deformed/crossed beaks (Galligan and Kleindorfer Reference Galligan and Kleindorfer2009, Kleindorfer and Sulloway Reference Kleindorfer and Sulloway2016, Fessl et al. Reference Fessl, Heimpel, Causton and Parker2018). Overall fitness will be negatively impacted in birds with deformed beaks (Kleindorfer and Sulloway Reference Kleindorfer and Sulloway2016) as these birds may have problems feeding, preening and singing. Darwin’s Finches are songbirds and beak shape is associated with song characteristics and assortative mating (Christensen et al. Reference Christensen, Kleindorfer and Robertson2006). Thus a change of naris size due to parasitism could have carry-over mate choice effects with possible individual- and population-level effects (Custance Reference Custance2015).
Birds worldwide are vulnerable to nest parasitism
We focused on the effects of Philornis on small and declining populations of Neotropical birds but parasitic nest flies occur worldwide. Other dipterans whose larvae are obligate parasites on nestling birds include members of the families Calliphoridae (Protocalliphora), Muscidae (Passeromyia) and Neottiophilidae (Neottiophilum). The genus Protocalliphora has a Holarctic distribution that includes North America, Nearctic Mexico, Palearctic Europe, North Africa and temperate Asia, with 26 described species (Sabrosky et al. Reference Sabrosky, Bennett and Whitworth1989). The genus Passeromyia occurs in Asia, South Africa, Australia, and the West Pacific (Pont Reference Pont1974), and includes five species (Couri and Carvalho Reference Couri and Carvalho2003). The genus Neottiophilum is Palearctic, with N. praestum being the only described member of the family (Owen Reference Owen1957). While these nest parasites collectively attack a variety of host species, the majority of host records are within the Passeriformes (Little Reference Little, Atkinson, Thomas and Hunter2008).
Two Canadian studies evaluated the effect of human-induced land disturbance on parasitism of Tree Swallows Tachycineta bicolor by Protocalliphora flies and are therefore relevant to our discussion. The first study compared the prevalence and intensity of Protocalliphora sialia, P. bennetti and P. braueri parasitism on Tree Swallows in a site disturbed due to oil sand mining in Alberta versus a control (undisturbed) site. Nests built on the disturbed wetlands were more heavily parasitised (harboured 60–72% more larvae) than control nests and infected nestlings presented reduced growth on the oil-sands-impacted wetlands than in the control site (Gentes et al. Reference Gentes, Whitworth, Waldner, Fenton and Smits2007). This case study shows how habitat destruction resulted in higher parasite infestation of nestlings by Protocalliphora spp. The second study followed the tri-trophic interactions among the same Tree Swallow species, its Protocalliphora parasites and their Nasonia parasitoid wasps along a gradient of agricultural intensification. The number of swallow fledglings, the abundance of P. sialia, and the level of Nasonia wasp parasitism were all negatively affected by the habitat loss, fragmentation and degradation associated with the intensification of agricultural practices (Daoust et al. Reference Daoust, Bélisle, Savage, Robillard, Baeta and Brodeur2012). In this case however, lower fledging rate in the presence of human disturbance could not be attributed to nest parasitism.
The effect of Passeromyia flies on rare bird hosts was investigated in Tasmania, where the endemic and ‘Endangered’ Forty-spotted Pardalotes Pardalotus quadragintus are restricted to isolated populations, with approximately 1,500 individuals left in the wild due to past forest clearing and fragmentation (BirdLife International 2017). This species inhabits second-growth forest where nesting cavities are limited and individuals are thus forced to compete aggressively with the Striated Pardalote P. substriatus for cavities (Edworthy Reference Edworthy2016a). In 2012, larvae of the endemic Passeromyia longicornis were found parasitising both pardalote species. Prevalence of P. longicornis in nests of Forty-spotted Pardalotes was 87% during a study spanning three breeding seasons. Nestling mortality in nests harbouring P. longicornis larvae was 85%, highlighting the detrimental effect of this fly on the already low Forty-spotted Pardalote population (Edworthy Reference Edworthy2016b).
Long-term management plans for bird populations subjected to nest parasitism
For bird species fitting into the declining-population paradigm that are under stress by habitat destruction or modification, the lack of high-quality nesting sites might influence offspring survival as the birds are forced to either build nests in sub-optimal habitats, increasing exposure to predators and/or parasites (as in the Tree Swallow example) or to fight for nest sites (as discussed for the Forty-spotted Pardalote), or a combination of both. Vertical habitat availability may be another key factor in species persistence, especially in range-restricted ones impacted by habitat loss at a vertical scale. Intensive agriculture in the highlands of Floreana Island in Galápagos leads to shorter-statured Scalesia trees than those on Santa Cruz Island where agricultural practices do not occur directly in Scalesia habitat. The highest nesting bird species in the Scalesia forest of Floreana Island (the Medium Tree Finch) sustained the most P. downsi larvae, but such a pattern was not observed on the highest nesting bird species on Santa Cruz Island (Peters and Kleindorfer Reference Peters and Kleindorfer2015). One study in central Argentina determined the drivers of Philornis parasite abundance at the microhabitat and community levels. In this case nest height had no effect on brood infestation but there was a strong reduction in mean larval abundance as the average forest height increased (except in forests dominated by exotic species). These findings suggest that for Philornis torquans in this case, what matters is not the height at which the hosts are but rather the microenvironment associated with differential forest height (Manzoli et al. Reference Manzoli, Antoniazzi, Saravia, Silvestri, Rorhmann and Beldomenico2013).
Still very little is known on how nest parasites locate hosts and how they survive dry seasons in highly seasonal habitats (Loye and Carroll Reference Loye and Carroll1998, Fessl et al. Reference Fessl, Heimpel, Causton and Parker2018). It is imperative that more field studies worldwide determine the presence of nest parasites in bird populations with some extinction risk in order to take action in time if needed. Nests of already small and declining bird populations should be examined first. Mitigating actions might include manually removing larvae from nestlings, applying a mild insecticide to nests to kill the fly larvae (Cristinacce et al. Reference Cristinacce, Handschuh, Switzer, Cole, Tatayah, Jones and Bell2009, Knutie et al. Reference Knutie, McNew, Bartlow, Vargas and Clayton2014), and the head-starting technique (Cristinacce et al. Reference Cristinacce, Ladkoo, Switzer, Jordan, Vencatasamy, de Ravel Koenig, Jones and Bell2008). For invasive nest parasites like P. downsi in Galápagos, the introduction of specialised biological control agents (Bulgarella et al. Reference Bulgarella, Quiroga, Boulton, Ramírez, Moon, Causton and Heimpel2017, Boulton and Heimpel Reference Boulton, Heimpel, Van Driesche and Reardon2017, Delvare et al. Reference Delvare, Heimpel, Baur, Chadee, Martinez and Knutie2017, Heimpel Reference Heimpel2017, Boulton et al. in press) or sterile male release may be feasible management options (Dudaniec et al. Reference Dudaniec, Gardner and Kleindorfer2010, Lahuatte et al. Reference Lahuatte, Lincango, Heimpel and Causton2016; Fessl et al. Reference Fessl, Heimpel, Causton and Parker2018).
By focusing on these six cases of Philornis parasitism on small, declining or isolated bird populations in the Neotropics, we aimed to highlight how sensitive these particular populations can be to dipteran nest parasites and how parasites can lead to imminent extinction. Philornis parasitism is just one case of nest parasites affecting bird species worldwide. For this reason, it is crucial to learn more about nest parasite-host behaviour, their general biology and their interactions before bird populations become at risk and incorporate this knowledge in bird conservation programmes. It is our intention to make researchers that are directly or indirectly involved in bird conservation aware of the implications of any conservation policy on the general health of bird populations and to highlight that parasitism is one of the many determinants of population well-being (Scott Reference Scott1988). Biodiversity research needs more boots on the ground (Wilson Reference Wilson2017).
Acknowledgements
We thank Dr Dusti Becker and Mauricio Torres for sharing the results of their fieldwork in Ecuador, and Dr Bradley J. Sinclair from the Canadian National Collection of Insects and Ottawa Plant Laboratory, Canada, for help identifying the fly puparia found in mainland Ecuador. For providing photographs for Figure 1, we thank Francesca Cunninghame, Berton Harris, Thomas Hayes, Sonia Kleindorfer and Julio C. Gallardo. We thank Francesca Cunninghame, Birgit Fessl, Charlotte Causton and Ricardo Palma for their useful comments that greatly improved our original manuscript. Sonia Kleindorfer and an anonymous reviewer provided helpful comments during the peer-review process.