Book contents
- Wildlife Disease Ecology
- Ecological Reviews
- Wildlife Disease Ecology
- Copyright page
- Contents
- Contributors
- Preface: Wildlife Disease Ecology
- Glossary of Terms
- Part I Understanding within-host processes
- Part II Understanding between-host processes
- Part III Understanding wildlife disease ecology at the community and landscape level
- Index
- Plate Section (PDF Only)
- References
Part III - Understanding wildlife disease ecology at the community and landscape level
Published online by Cambridge University Press: 28 October 2019
- Wildlife Disease Ecology
- Ecological Reviews
- Wildlife Disease Ecology
- Copyright page
- Contents
- Contributors
- Preface: Wildlife Disease Ecology
- Glossary of Terms
- Part I Understanding within-host processes
- Part II Understanding between-host processes
- Part III Understanding wildlife disease ecology at the community and landscape level
- Index
- Plate Section (PDF Only)
- References
Summary
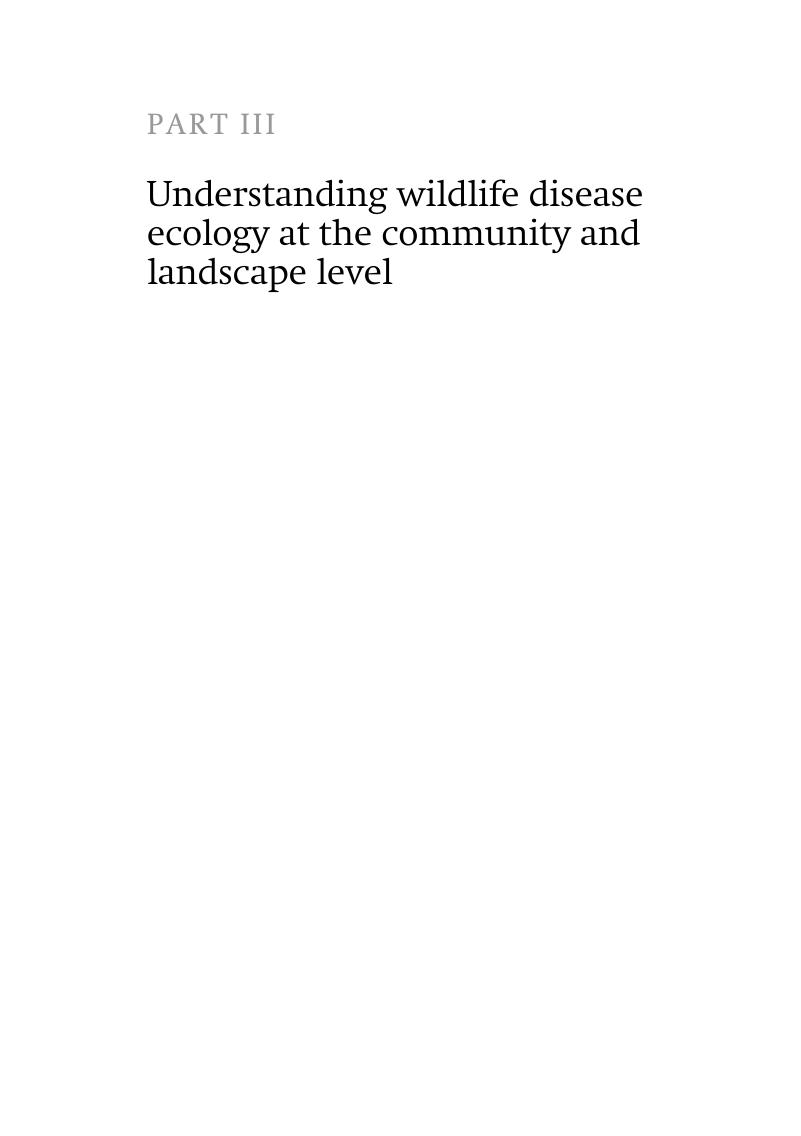
Keywords
- Type
- Chapter
- Information
- Wildlife Disease EcologyLinking Theory to Data and Application, pp. 427 - 643Publisher: Cambridge University PressPrint publication year: 2019