Book contents
- Escaping From PredatorsAn Integrative View of Escape Decisions
- Escaping From Predators
- Copyright page
- Dedication
- Contents
- Contributors
- Foreword
- Book part
- Part I Overview and behaviors preceding and following initiation of escape
- Part II Escape and refuge use: theory and findings for major taxonomic groups
- IIa Escape theory
- IIb Escape decisions prior to pursuit
- IIc Escape trajectories and strategies during pursuit
- 8 Prey behaviors during fleeing: escape trajectories, signaling, and sensory defenses
- IId Refuge use
- Part III Related behaviors and other factors influencing escape
- Part IV The application and study of escape
- Index
- References
8 - Prey behaviors during fleeing: escape trajectories, signaling, and sensory defenses
from IIc - Escape trajectories and strategies during pursuit
Published online by Cambridge University Press: 05 June 2015
- Escaping From PredatorsAn Integrative View of Escape Decisions
- Escaping From Predators
- Copyright page
- Dedication
- Contents
- Contributors
- Foreword
- Book part
- Part I Overview and behaviors preceding and following initiation of escape
- Part II Escape and refuge use: theory and findings for major taxonomic groups
- IIa Escape theory
- IIb Escape decisions prior to pursuit
- IIc Escape trajectories and strategies during pursuit
- 8 Prey behaviors during fleeing: escape trajectories, signaling, and sensory defenses
- IId Refuge use
- Part III Related behaviors and other factors influencing escape
- Part IV The application and study of escape
- Index
- References
Summary
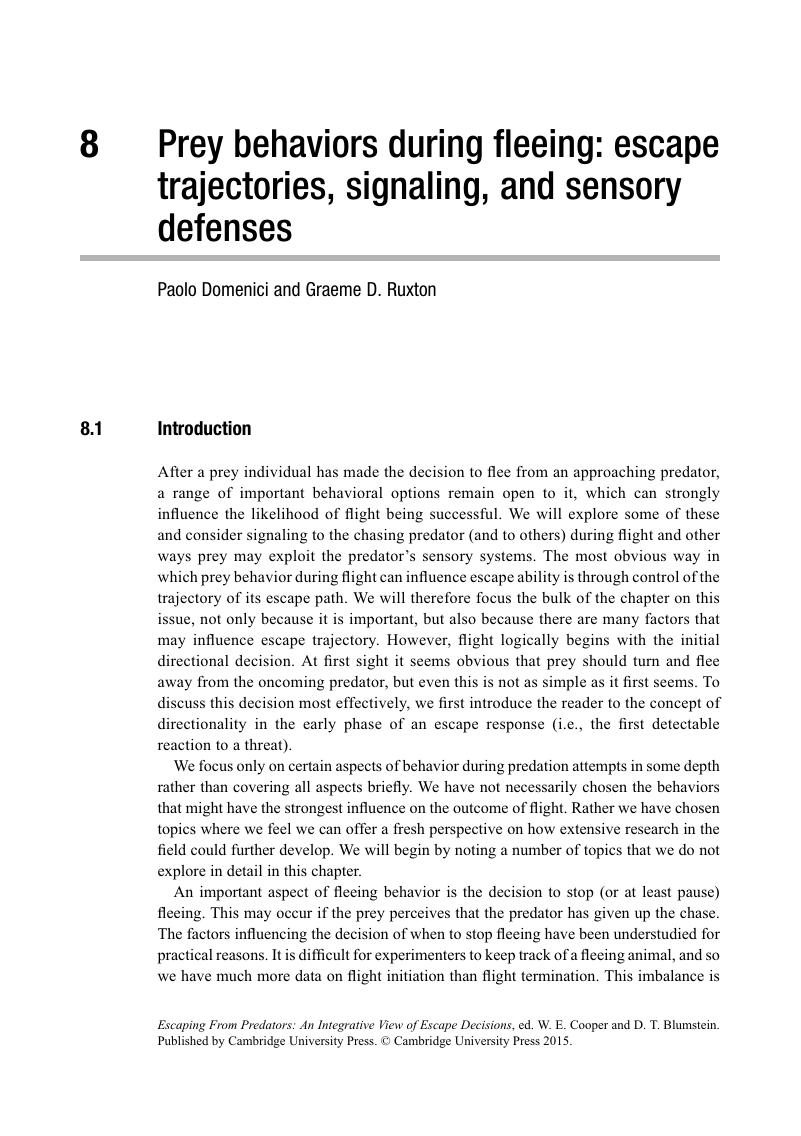
- Type
- Chapter
- Information
- Escaping From PredatorsAn Integrative View of Escape Decisions, pp. 199 - 224Publisher: Cambridge University PressPrint publication year: 2015
References
- 9
- Cited by