Book contents
- Coarse Grained Simulation and Turbulent Mixing
- Coarse Grained Simulation and Turbulent Mixing
- Copyright page
- Dedication
- Contents
- Contributors
- Preface
- Prologue
- Part I Fundamentals
- 1 Proof of Concept
- 2 A Minimum Turbulence State for Coarse Grained Simulation
- 3 Finite Scale Theory
- 4 Material Conservation of Passive Scalar Mixing in Finite Scale Navier Stokes Fluid Turbulence
- Part II Challenges
- Part III Complex Mixing Consequences
- Index
- Plate section
- References
2 - A Minimum Turbulence State for Coarse Grained Simulation
from Part I - Fundamentals
Published online by Cambridge University Press: 05 June 2016
- Coarse Grained Simulation and Turbulent Mixing
- Coarse Grained Simulation and Turbulent Mixing
- Copyright page
- Dedication
- Contents
- Contributors
- Preface
- Prologue
- Part I Fundamentals
- 1 Proof of Concept
- 2 A Minimum Turbulence State for Coarse Grained Simulation
- 3 Finite Scale Theory
- 4 Material Conservation of Passive Scalar Mixing in Finite Scale Navier Stokes Fluid Turbulence
- Part II Challenges
- Part III Complex Mixing Consequences
- Index
- Plate section
- References
Summary
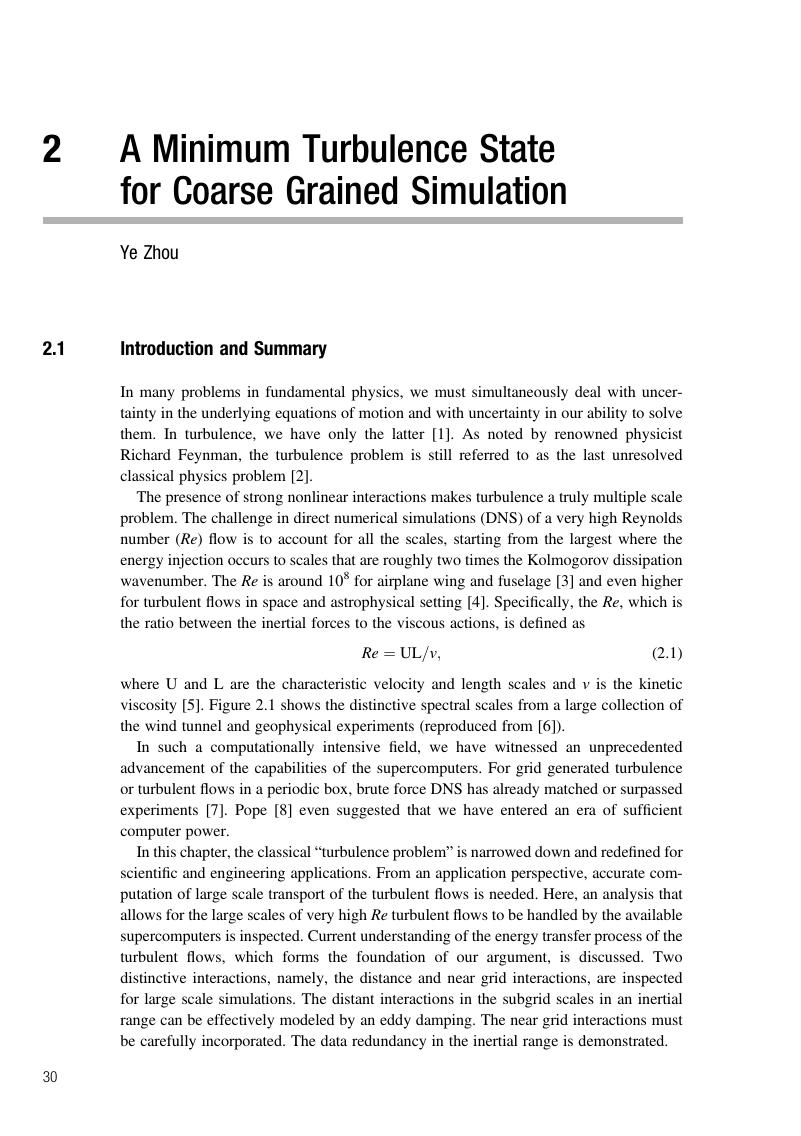
- Type
- Chapter
- Information
- Coarse Grained Simulation and Turbulent Mixing , pp. 30 - 47Publisher: Cambridge University PressPrint publication year: 2016