Book contents
- Frontmatter
- Contents
- Preface
- List of Symbols
- 1 Thermodynamics and the Earth system
- 2 Energy and entropy
- 3 The first and second law of thermodynamics
- 4 Thermodynamic limits
- 5 Dynamics, structures, and maximization
- 6 Radiation
- 7 Motion
- 8 Hydrologic cycling
- 9 Geochemical cycling
- 10 Land
- 11 Human activity
- 12 The thermodynamic Earth system
- Glossary
- References
- Index
- References
References
Published online by Cambridge University Press: 05 March 2016
- Frontmatter
- Contents
- Preface
- List of Symbols
- 1 Thermodynamics and the Earth system
- 2 Energy and entropy
- 3 The first and second law of thermodynamics
- 4 Thermodynamic limits
- 5 Dynamics, structures, and maximization
- 6 Radiation
- 7 Motion
- 8 Hydrologic cycling
- 9 Geochemical cycling
- 10 Land
- 11 Human activity
- 12 The thermodynamic Earth system
- Glossary
- References
- Index
- References
Summary
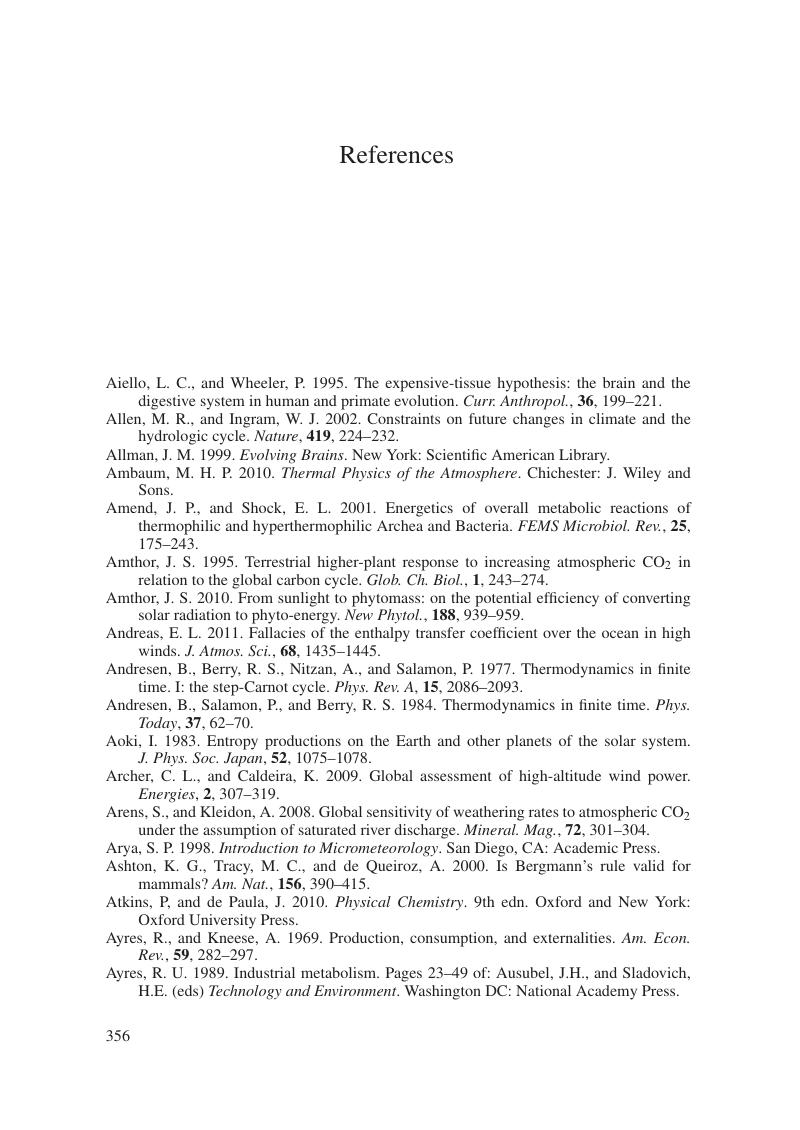
- Type
- Chapter
- Information
- Thermodynamic Foundations of the Earth System , pp. 356 - 374Publisher: Cambridge University PressPrint publication year: 2016