Book contents
- Genome Editing and Engineering
- Genome Editing and Engineering
- Copyright page
- Dedication
- Contents
- Contributors
- Foreword
- Preface
- Part I Biology of Endonucleases (Zinc-Finger Nuclease, TALENs and CRISPRs) and Regulatory Networks
- Part II Genome Editing in Model Organisms
- Part III Technology Development and Screening
- Part IV Genome Editing in Stem Cells and Regenerative Biology
- 16 Targeted Genome Editing Using Nuclease-assisted Vector Integration
- 17 Genome Engineering Using Sleeping Beauty Transposition in Vertebrates
- 18 Genome Editing of Pluripotent Stem Cells
- 19 Principles for Targeting Adult Tissue Stem Cells to Achieve Durable Gene and Gene Editing Therapies
- 20 Therapeutic Genome Editing in Human Hematopoietic Stem and Progenitor Cells
- Part V Genome Editing in Disease Biology
- Part VI Legal (Intellectual Property) and Bioethical Issues of Genome Editing
- Index
- Plate Section (PDF Only)
- References
16 - Targeted Genome Editing Using Nuclease-assisted Vector Integration
from Part IV - Genome Editing in Stem Cells and Regenerative Biology
Published online by Cambridge University Press: 30 July 2018
- Genome Editing and Engineering
- Genome Editing and Engineering
- Copyright page
- Dedication
- Contents
- Contributors
- Foreword
- Preface
- Part I Biology of Endonucleases (Zinc-Finger Nuclease, TALENs and CRISPRs) and Regulatory Networks
- Part II Genome Editing in Model Organisms
- Part III Technology Development and Screening
- Part IV Genome Editing in Stem Cells and Regenerative Biology
- 16 Targeted Genome Editing Using Nuclease-assisted Vector Integration
- 17 Genome Engineering Using Sleeping Beauty Transposition in Vertebrates
- 18 Genome Editing of Pluripotent Stem Cells
- 19 Principles for Targeting Adult Tissue Stem Cells to Achieve Durable Gene and Gene Editing Therapies
- 20 Therapeutic Genome Editing in Human Hematopoietic Stem and Progenitor Cells
- Part V Genome Editing in Disease Biology
- Part VI Legal (Intellectual Property) and Bioethical Issues of Genome Editing
- Index
- Plate Section (PDF Only)
- References
Summary
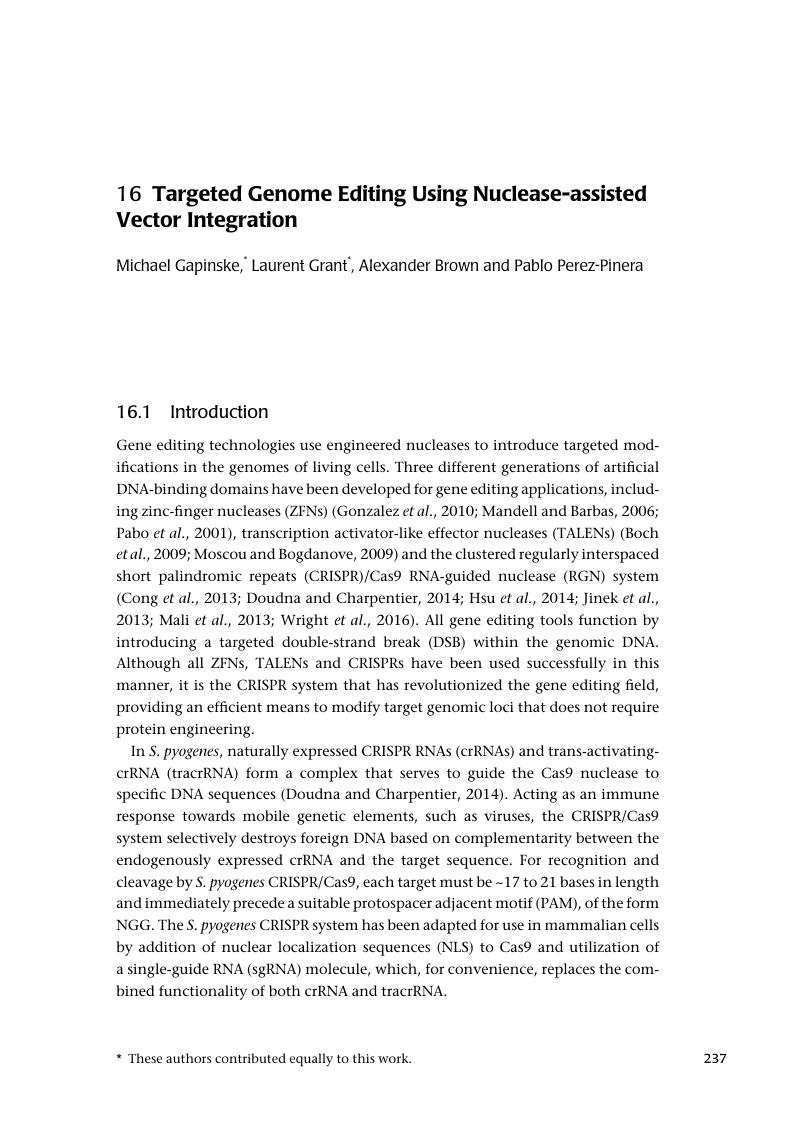
- Type
- Chapter
- Information
- Genome Editing and EngineeringFrom TALENs, ZFNs and CRISPRs to Molecular Surgery, pp. 237 - 248Publisher: Cambridge University PressPrint publication year: 2018