Book contents
- Fundamentals, Properties, and Applications of Polymer Nanocomposites
- Fundamentals, Properties, and Applications of Polymer Nanocomposites
- Copyright page
- Dedication
- Contents
- Preface
- Part One Fundamentals, Processing, and Characterization
- Part Two Multifunctional Properties and Applications
- Part Three Concerns and Outlook
- Index
- References
Part One - Fundamentals, Processing, and Characterization
Published online by Cambridge University Press: 27 January 2017
- Fundamentals, Properties, and Applications of Polymer Nanocomposites
- Fundamentals, Properties, and Applications of Polymer Nanocomposites
- Copyright page
- Dedication
- Contents
- Preface
- Part One Fundamentals, Processing, and Characterization
- Part Two Multifunctional Properties and Applications
- Part Three Concerns and Outlook
- Index
- References
Summary
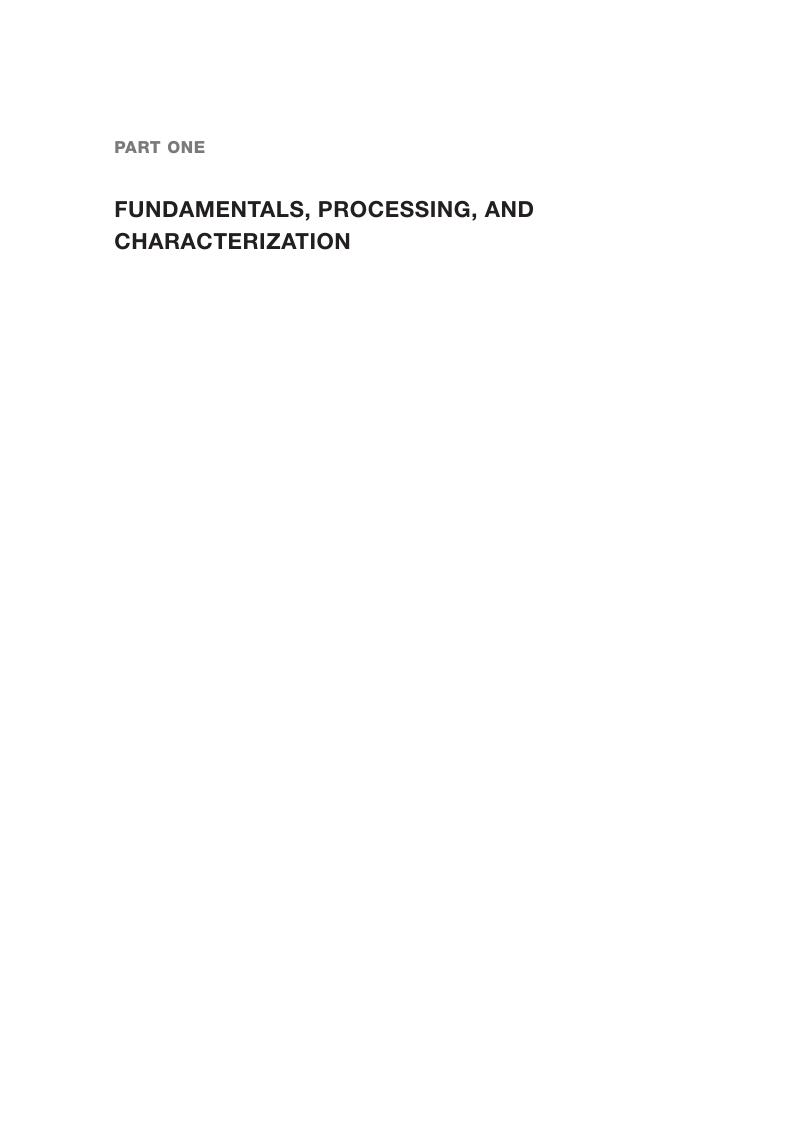
- Type
- Chapter
- Information
- Publisher: Cambridge University PressPrint publication year: 2016