Book contents
- Frontmatter
- Contents
- List of Abbreviations
- List of Symbols
- 1 Perfusion Mammalian Cell Culture for Recombinant Protein Manufacturing
- 2 Perfusion Bioreactors:The Set-Up and Process Characterisation
- 3 Scale-Down Models and Sensors for Process Development
- 4 Design and Optimisation of Mammalian Cell Perfusion Cultures
- 5 Clinical- and Commercial-Scale Reactors
- 6 Mechanistic and Statistical Modelling of Bioprocesses
- References
- Index
- References
References
Published online by Cambridge University Press: 17 July 2020
- Frontmatter
- Contents
- List of Abbreviations
- List of Symbols
- 1 Perfusion Mammalian Cell Culture for Recombinant Protein Manufacturing
- 2 Perfusion Bioreactors:The Set-Up and Process Characterisation
- 3 Scale-Down Models and Sensors for Process Development
- 4 Design and Optimisation of Mammalian Cell Perfusion Cultures
- 5 Clinical- and Commercial-Scale Reactors
- 6 Mechanistic and Statistical Modelling of Bioprocesses
- References
- Index
- References
Summary
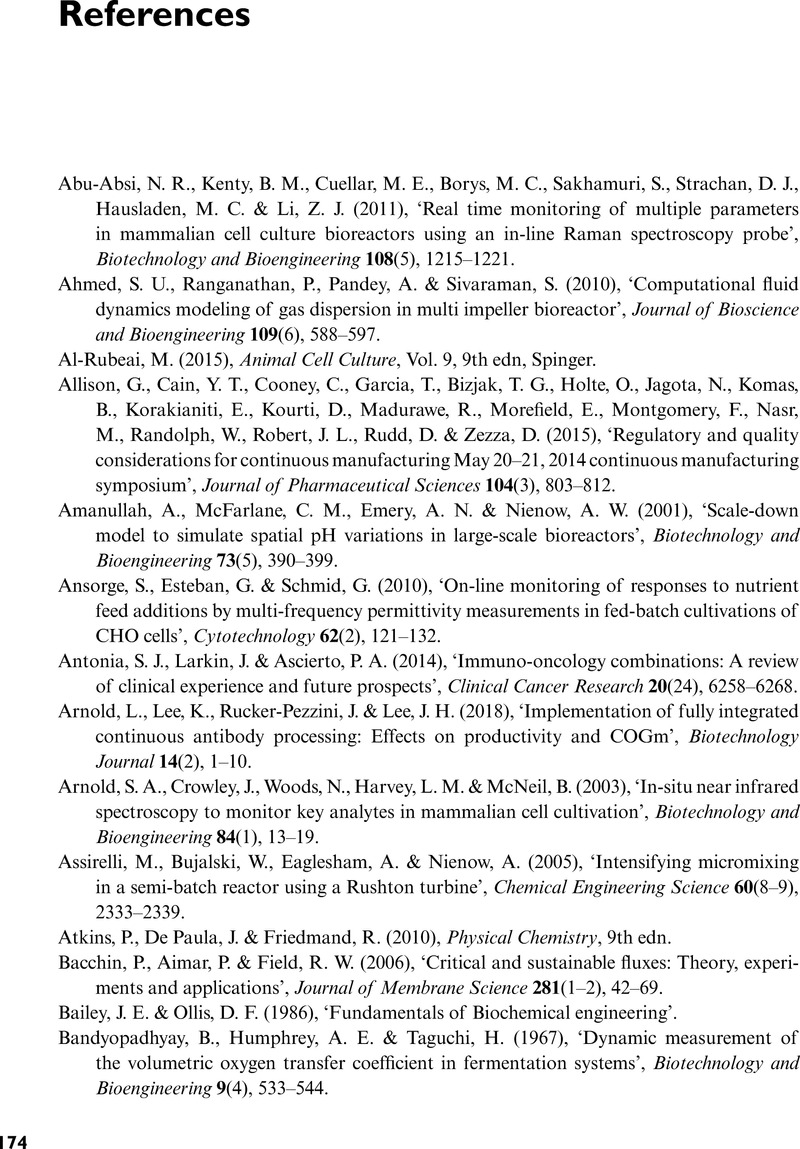
- Type
- Chapter
- Information
- Perfusion Cell Culture Processes for BiopharmaceuticalsProcess Development, Design, and Scale-up, pp. 174 - 197Publisher: Cambridge University PressPrint publication year: 2020