The maintenance of skeletal muscle mass and function with advancing age is fundamental to metabolic health across the lifespan(Reference Wolfe1), and is primarily achieved through the prevention or improved management of muscle wasting, termed sarcopenia. In essence, sarcopenia is defined as a reduction in muscle mass, strength and function(Reference Cruz-Jentoft, Bahat and Bauer2), and is prevalent in older adults(Reference Shafiee, Keshtkar and Soltani3,Reference Mayhew, Amog and Phillips4) . Skeletal muscle also acts as the primary site for insulin-stimulated glucose uptake, and therefore the dysregulation of this body tissue contributes to the development of metabolic syndrome, type II diabetes and increased risk of CVD(Reference Stump, Henriksen and Wei5). Sarcopenia also underpins frailty and the decline in functional capacity that compromises quality of life(Reference Beaudart, Zaaria and Pasleau6), and is associated with a range of clinical disorders, such as osteoporosis and obesity(Reference Kuh, Karunananthan and Bergman7). Although the aetiology of sarcopenia is clearly multifactorial and not fully understood(Reference Morley8), key underlying factors include morphological changes in skeletal muscle(Reference Patel, White and Westbury9), the loss of motor units(Reference Piasecki, Ireland and Stashuk10), physical inactivity(Reference Saoi, Li and McGlory11) and, particularly prudent to this review, dietary protein and energy deficiencies(Reference Murphy and Roche12).
The combination of adequate dietary protein intake and muscle loading (i.e. resistance) exercise serves as the cornerstone for the management of sarcopenia in both institutionalised and non-institutionalised older adults(Reference Murphy and Roche12–Reference Baum, Kim and Wolfe17). Another key component of an effective countermeasure for sarcopenia includes adequate energy intake(Reference Morley, Argiles and Evans18), which is typically reduced with ageing(Reference Johnson, Shannon and Matu19). Although protein-energy malnutrition and undernutrition are often associated with institutionalised settings(Reference Elia20–Reference Kaiser, Bauer and Rämsch22) wherein the majority of residents are older adults, protein-energy undernutrition is also prevalent in community-dwelling older populations. According to recent reports, about 8 % of community-dwelling older adults are classified as malnourished and 35 % are at risk of malnutrition(Reference Rist, Miles and Karimi23), whereas 66 % of those admitted to a hospital are at risk of malnutrition(Reference Geurden, Franck and Weyler24). Dependent on the degree of deficiency, protein-energy undernutrition positions older adults at greater risk of other co-morbidities and mortality(Reference Sullivan, Bopp and Roberson25,Reference Sullivan and Walls26) . Given that immune cell function is dependent on energy availability and amino acid provision, protein-energy undernutrition is also implicated in impaired immunity and increased susceptibility to viral infections with advancing age(Reference Alam, Almajwal and Alam27). Thus, older adults should ensure adequate provision of dietary protein and energy to maintain good health.
Alongside the importance of consuming sufficient daily protein intake, the effective management of sarcopenia also depends on the quantity and quality of protein consumed on a more refined ‘per meal’ basis(Reference Murphy, Oikawa and Phillips28). In this regard, older adults require a higher per meal dose of protein (about 0⋅4 g/kg body mass) compared to their younger counterparts (about 0⋅24 g/kg body mass) to maximally stimulate postprandial muscle protein synthesis (MPS) rates(Reference Moore, Churchward-Venne and Witard29). Protein quality is traditionally assessed by the protein digestibility-corrected amino acid score(Reference Millward, Layman and Tomé30,Reference Phillips31) , whereby the digestion and absorption kinetics of essential amino acids (EAA) determine the nutritional quality of a protein source. A more contemporary, and increasingly preferred, tool for assessing protein quality is the digestible indispensable amino acid score since the protein digestibility-corrected amino acid score tends to overestimate the value of lower quality proteins(Reference Phillips31). Of all the EAA, leucine plays a prominent role in regulating muscle metabolism via direct action on the mammalian target of rapamycin (mTOR) complex 1 (mTORC1) signalling pathway that switches on MPS(Reference Atherton, Smith and Etheridge32–Reference Moberg, Apró and Ekblom34), an effect that is potentiated by the other EAA(Reference Moberg, Apró and Ekblom34). At the metabolic level, the maintenance of skeletal muscle mass is contingent on muscle protein balance, whereby the rates of muscle protein breakdown are offset against the rates of MPS(Reference Trommelen, Betz and van Loon35,Reference Murton36) . Hence, on a per meal basis, the consumption of adequate, high-quality protein sources that comprise the full complement of EAA represents an intuitive strategy for the management of sarcopenia. Moreover, there is extensive evidence that combining protein supplementation with resistance exercise training exhibits a synergistic effect on skeletal muscle mass, strength and functional outcomes in older adults(Reference Cermak, Res and Groot37,Reference Morton, Murphy and McKellar38) , with exercise sensitising skeletal muscle to the anabolic action of EAA(Reference Moberg, Apró and Ekblom34,Reference Lees, Wilson and Webb39,Reference Rasmussen, Tipton and Miller40) . On this basis, lifestyle strategies designed to combat sarcopenia and promote skeletal muscle health in older adults require a holistic approach encompassing both exercise and protein nutrition interventions (Fig. 1).
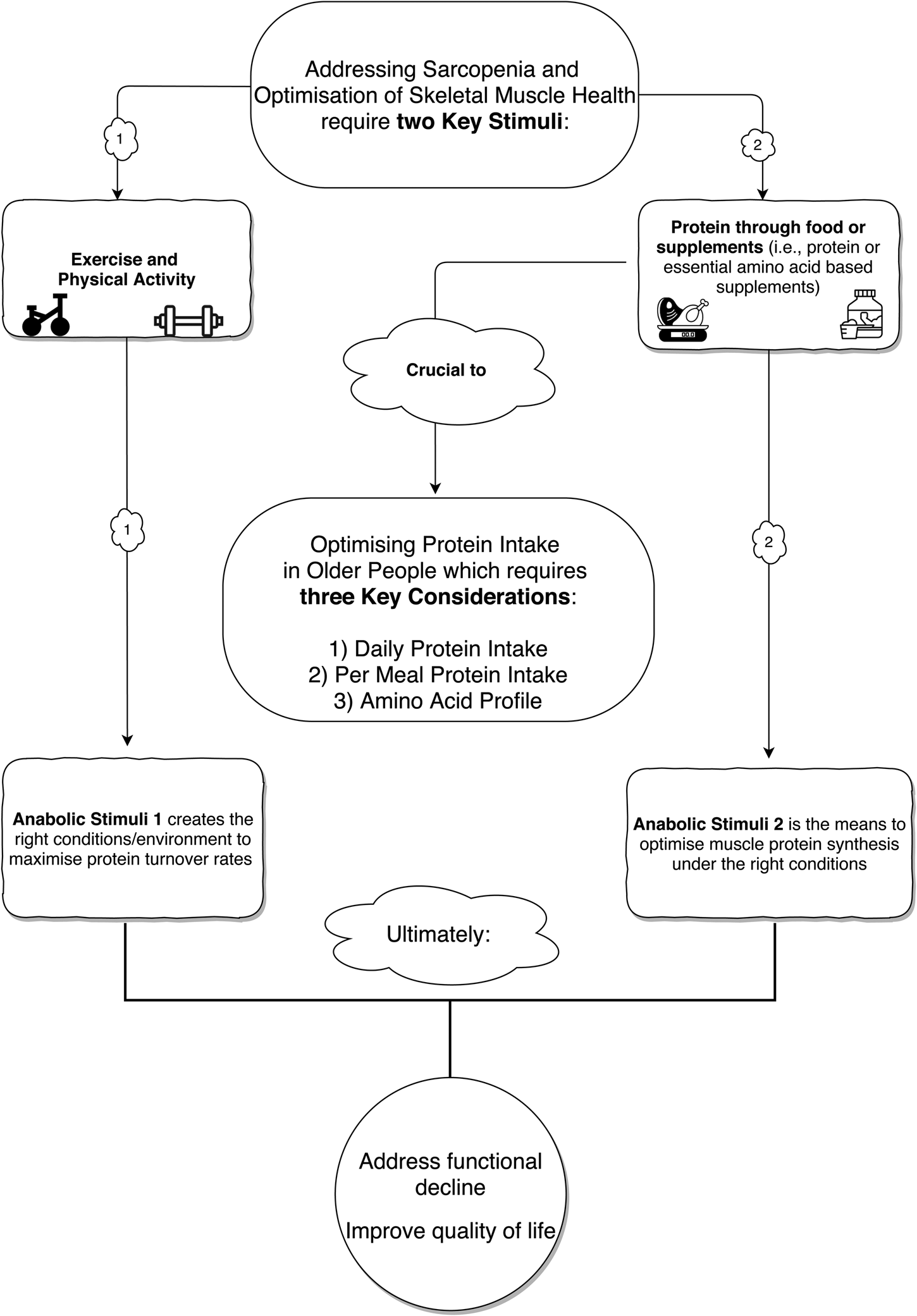
Fig. 1. A holistic approach to addressing the challenge of age-associated sarcopenia and optimising skeletal muscle health with reference to dietary protein intake (food and supplementation) and physical activity/exercise.
Protein is the most satiating of all macronutrients(Reference Poppitt, McCormack and Buffenstein41). Given that older adults commonly experience a reduction in appetite(Reference Johnson, Shannon and Matu19), difficulties with chewing, dysphagia(Reference Hudson, Daubert and Mills42) and suppression of taste and smell(Reference Landi, Calvani and Tosato43), it may be considered paradoxical to recommend increased protein intakes, potentially at the expense of overall energy intake. Moreover, ageing is associated with reductions in gastric emptying and the splanchnic uptake of amino acids(Reference Landi, Calvani and Tosato43) that can be further compromised depending on the type of protein ingested(Reference Boirie, Dangin and Gachon44). As such, older adults are presented with additional challenges in terms of achieving higher daily and per meal protein intakes, which tend to be particularly low at breakfast and lunch(Reference Tieland, Borgonjen-Van den Berg and Van Loon45). Thus, it is imperative that interventions targeted at increasing total dietary protein intake are not counter-productive in exacerbating energy deficiencies in older adults at risk of negative energy balance, or who would benefit from increasing energy intake.
The aims of this review are 2-fold. First, to critically evaluate the existing evidence base that has explored the efficacy of EAA ingestion as a strategy to increase daily and per meal dietary protein intakes, without compromising intake of other nutrients (macro- and micro-nutrients) in older adults. Secondly, to critically evaluate findings from experimental studies investigating the impact of EAA supplementation on acute (i.e. muscle protein turnover) and chronic (i.e. body composition, strength and muscle function) musculoskeletal health outcomes in older adults, with a view to advancing evidence-based practical guidelines for the management of sarcopenia. By way of introduction, we provide a brief overview regarding the evolution of dietary protein recommendations in the context of managing sarcopenia.
Dietary protein intake and sarcopenia
Re-evaluating the protein RDA in older adults
There is general consensus among the scientific community that daily protein intakes should exceed the current RDA (0⋅8 g/kg body mass per day) for the management of sarcopenia(Reference Lang, Streeper and Cawthon13,Reference Naseeb and Volpe15–Reference Baum, Kim and Wolfe17) and preservation of muscle mass and function in older adults(Reference Bauer, Biolo and Cederholm46–Reference Phillips, Chevalier and Leidy50). Consistent with this notion, achieving the current RDA may result in a daily protein intake below the acceptable macronutrient distribution range of about 15–35 %(Reference Wolfe, Miller and Miller51,Reference Manore52) . Multiple lines of evidence(Reference Deutz, Bauer and Barazzoni48,Reference Kim, Schutzler and Schrader53–Reference Mitchell, Milan and Mitchell55) substantiate the recommendation to increase protein intakes above the RDA in older adults. First, although cause and effect cannot be established based on observational studies, Houston et al.(Reference Houston, Nicklas and Ding54) documented that healthy older adults consuming a protein intake in excess of the protein RDA were associated with an attenuated decline in muscle mass over a 3 year follow-up period. However, older adults that consumed a protein intake only marginally in excess of the RDA (i.e. up to 1⋅1 g/kg per day) still suffered a decline in muscle mass. Secondly, a recent carefully controlled study by Mitchell et al.(Reference Mitchell, Milan and Mitchell55) demonstrated that increasing protein intake to twice the RDA (i.e. 1⋅6 g/kg per day) resulted in significant gains in lean tissue mass in healthy older men. Furthermore, older individuals have been shown to require a higher per meal protein intake because they are less sensitive to anabolic stimuli such as protein; a concept termed skeletal muscle ‘anabolic resistance’(Reference Rennie56,Reference Breen and Phillips57) . Short-term periods of reduced physical activity or muscle disuse (i.e. bed rest or limb immobilisation) have been shown to induce anabolic resistance in older individuals, as indicated by reduced skeletal muscle amino acid transporter expression, impaired mTORC1 signalling, and blunted postprandial MPS rates in response to EAA(Reference Breen, Stokes and Churchward-Venne58–Reference Wall, Gorissen and Pennings60). In this regard, and when expressed relative to body mass, older adults require a protein dose of about 0⋅4 g/kg body mass per meal (i.e. about 30 g protein for an average male) for the maximal postprandial stimulation of MPS, which is about 67 % higher than the relative protein dose required for younger adults(Reference Moore, Churchward-Venne and Witard29). Accordingly, reputable international expert groups such as the European Society for Clinical Nutrition and Metabolism have called for higher protein intakes in older adults(Reference Deutz, Bauer and Barazzoni48) with recommendations set in relation to the current protein RDA: a 25–50 % increase for healthy individuals, a 50–90 % increase those suffering from acute or chronic disease, and greater than 50 % increase above the RDA for those experiencing severe illness or injury. Notwithstanding these latest guidelines, a large proportion of older adults fail to meet the existing RDA in terms of habitual protein intake. For example, in a large cohort of older adults who participated in the National Health and Nutrition Examination Survey, over 30 % failed to meet the current protein RDA(Reference Krok-Schoen, Archdeacon Price and Luo61). This observation highlights the practical issues associated with older adults successfully meeting the recommended protein intakes for the management of sarcopenia.
Rationale for dietary protein supplementation in older adults
Achieving a protein intake in excess of the RDA and in accordance with the European Society for Clinical Nutrition and Metabolism guidelines(Reference Deutz, Bauer and Barazzoni48) presents a profound challenge for older adults, given that this population commonly suffers from acute or chronic illness. This concern is exemplified by the high prevalence of malnutrition and anorexia in these compromised populations(Reference Rist, Miles and Karimi23,Reference Geurden, Franck and Weyler24,Reference Butterworth, Lees and Harlow62–Reference Cramer, Cruz-Jentoft and Landi64) . In theory, increasing daily protein intake to twice the RDA translates to an 80 kg older adult consuming about 130 g protein daily. Given that protein increases satiety(Reference Veldhorst, Smeets and Soenen65,Reference Mollahosseini, Shab-Bidar and Rahimi66) in a dose-dependent manner(Reference Veldhorst, Nieuwenhuizen and Hochstenbach-Waelen67,Reference Paddon-Jones and Leidy68) , and appetite is dysregulated in older adults(Reference Johnson, Shannon and Matu19), meeting this target daily protein intake is not pragmatic for all older adults. Therefore, strategies to increase protein intake either through whole food or nutritional supplementation should not be at the expense of other nutrients and/or contribute to a reduction in overall energy intake.
The recommendation to increase protein intake while simultaneously maintaining, and in many cases increasing energy intake, can present a ‘protein paradox’ (Fig. 2). In this regard, dietary supplementation strategies to increase protein intake may unintentionally result in partial energy redistribution(Reference Fiatarone Singh, Bernstein and Ryan63,Reference Cramer, Cruz-Jentoft and Landi64) , which may negatively affect both protein and energy intakes. A reduction in energy intake may be desirable for certain groups (i.e. individuals with obesity), however the focus of this review is on increasing protein intake in older adults without affecting their ability to maintain energy balance.
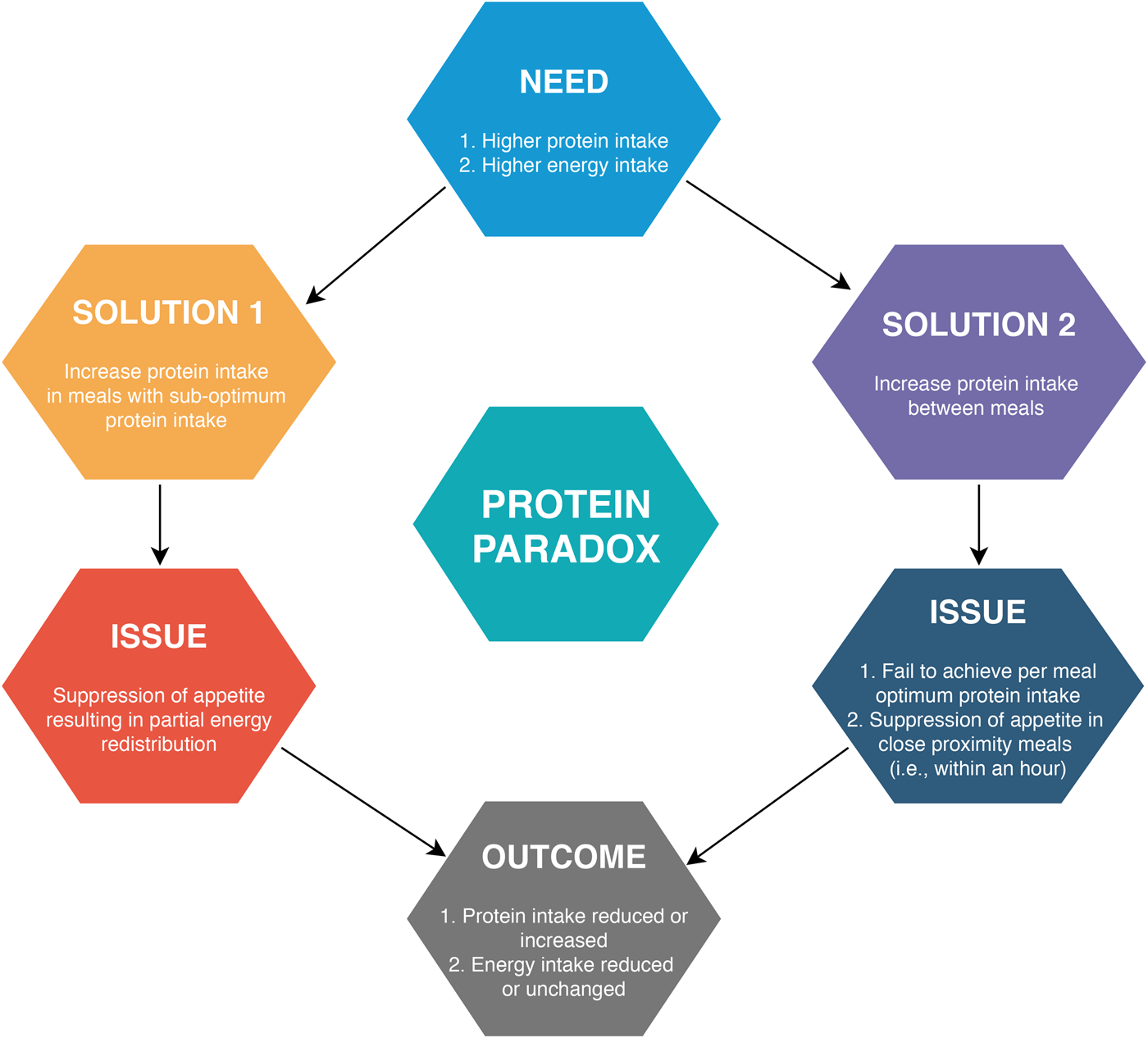
Fig. 2. A ‘protein paradox’ whereby increased protein intake through food or supplementation may not result in an increase in protein intake and compromise energy intake in older adults suffering from protein and energy deficiencies.
Protein supplementation alone or in combination with resistance exercise in older adults
Despite compelling proof-of-concept evidence(Reference Kim, Schutzler and Schrader53,Reference Yang, Breen and Burd69) from acute metabolic studies that increasing dietary protein intake may counter the age-related decline in muscle mass, the efficacy of chronic nutritional interventions to increase dietary protein intake is weak. This observation is particularly relevant when considering studies of protein-based supplementation in the absence of prescribed exercise training. Moreover, limited evidence exists regarding the benefits of protein supplementation v. placebo for maintaining muscle mass in healthy older adults(Reference Tieland, Franssen and Dullemeijer70). Multiple factors likely explain these surprising findings, including the duration of the intervention, habitual daily protein intake, and sample size. However, when combining the anabolic stimuli of protein supplementation and resistance exercise training, findings are more consistent in demonstrating improvements in outcome measurements of muscle health in older adults. The capacity of resistance exercise to sensitise muscle to the anabolic potential of dietary protein is primarily achieved through a timely supply of EAA(Reference Moberg, Apró and Ekblom34,Reference Lees, Wilson and Webb39,Reference Rasmussen, Tipton and Miller40) . Accordingly, a meta-analysis by Cermak et al.(Reference Cermak, Res and Groot37) revealed that gains in lean tissue mass were of greater magnitude in both younger and older adults when combining resistance training with protein supplementation v. resistance training alone. Consistent with this observation, Morton et al.(Reference Morton, Murphy and McKellar38) reported that protein supplementation augmented muscle growth during resistance training when habitual dietary protein intakes were, on average, below 1⋅6 g/kg body mass per day in younger adults. However, the impact of protein supplementation on muscle mass was reduced with advancing age. Interestingly, Cermak et al.(Reference Cermak, Res and Groot37) demonstrated similar increases in type II muscle fibre cross-sectional area in older adults following resistance training, irrespective of whether the study administered protein or a placebo. This observation is physiologically relevant, given that type II muscle fibres exhibit the greatest capacity for hypertrophy following resistance training(Reference Verdijk, Gleeson and Jonkers71), with studies consistently demonstrating smaller type II fibres in both healthy and frail older adults compared with younger adults(Reference Verdijk, Gleeson and Jonkers71–Reference Kosek, Kim and Petrella74). Moreover, the increased excitation threshold of type II fibres due to their greater innervation by large motor neurons(Reference Sale75) supports the utility of resistance training(Reference Häkkinen, Newton and Gordon76) as an exercise mode to recruit these large motor neurons and potentially minimise type II muscle fibre atrophy in older individuals. However, in practice, data from the Health Survey for England and Scotland (1994–2008, n 80 306) reported that only 3⋅4 % of individuals meet the strength-based exercise recommendations(Reference Stamatakis, Lee and Bennie77). Therefore, in addition to optimising protein intake, it is important for older adults to engage in strength-based exercise training to prolong independence, functional capacity and quality of life(Reference Liu and Latham78–Reference Krist, Dimeo and Keil80).
Protein quality and amino acid composition
The absolute and relative intake of dietary protein is an important consideration for supporting skeletal muscle hypertrophy. However, the quality of a given protein source is of additional importance for maintaining muscle mass in the context of healthy ageing and is defined by EAA content and digestibility of the ingested protein(Reference Millward, Layman and Tomé30,Reference Phillips31) . Moreover, protein quality as assessed using the digestible indispensable amino acid score accounts for each individual amino acid as a nutrient in its own right(Reference Phillips31). The amino acid composition of a given protein source can influence the extent and amplitude of postprandial MPS, and induce varying patterns of aminoacidaemia(Reference Franzke, Neubauer and Cameron-Smith81,Reference Traylor, Gorissen and Phillips82) . As previously outlined, the leucine content of a given protein source is particularly important in attenuating declines in muscle mass when consumed alongside other EAA(Reference Devries, McGlory and Bolster83). It follows that older adults require higher amounts of leucine to facilitate the maximal stimulation of MPS in the presence of other EAA(Reference Phillips, Chevalier and Leidy50). Therefore, it is important that older adults consume high-quality proteins(Reference Hamarsland, Laahne and Paulsen84) that provide a full complement of EAA for skeletal muscle health.
Optimising protein intake requires consideration of the following key points: (a) daily protein intake; (b) protein intake on a per meal basis and (c) protein quality as determined by a full and optimal complement of EAA. The broader role of EAA as key regulators of MPS is highlighted by Park et al.(Reference Park, Church and Azhar85) who compared the response of MPS to a low dose EAA mixture (3⋅2 g EAA; 2⋅4 g protein), a high EAA mixture (6⋅4 g EAA; 4⋅4 g protein) and an intact protein supplement containing 17⋅9 g whey. The combined ingestion of EAA with a low whey protein dose stimulated a greater anabolic response v. whey protein alone, a finding partially attributed to the rapid aminoacidaemia observed in the high EAA condition. This observation further supports the notion that provision of ample dietary EAA and leucine are necessary to support a skeletal muscle anabolic response in older adults. From a practical standpoint, the strategic nutritional supplementation with EAA and leucine alongside meals containing suboptimal protein content (i.e. breakfast and lunch) could assist older adults in achieving their per meal protein intake and EAA profile objectives. Nevertheless, it must also be noted that skeletal muscle is refractory to the anabolic effects of leucine during the postprandial ‘muscle-full’ period(Reference Atherton, Etheridge and Watt86,Reference Mitchell, Phillips and Hill87) . Therefore, in cases where older adults receive adequate high-quality protein with their main meals, it would be prudent that protein-based snacks or supplements are administered between meals when additional nutritional supplementation is required to reach their daily protein goal.
Effects of essential amino acid supplementation on dietary protein intake
In addition to the aforementioned nutritional plan considerations, the age-related anorexia and satiating properties of protein must be taken into account. Although it has been proposed that EAA are not satiating(Reference Ferrando, Paddon-Jones and Hays88–Reference Bukhari, Phillips and Wilkinson92), limited studies have investigated the acute(Reference Butterworth, Lees and Harlow62,Reference Ispoglou, Deighton and King93) or long-term impact of EAA supplementation on dietary intake(Reference Rondanelli, Opizzi and Antoniello91,Reference Ispoglou, White and Preston94) . Acute studies investigating the efficacy of EAA focus on the measurement of MPS under laboratory conditions where dietary intakes are strictly controlled(Reference Paddon-Jones, Sheffield-Moore and Aarsland90,Reference Bukhari, Phillips and Wilkinson92) , whereas longer term studies may(Reference Ferrando, Paddon-Jones and Hays88,Reference Rondanelli, Opizzi and Antoniello91,Reference Ispoglou, White and Preston94) or may not(Reference Dillon, Sheffield-Moore and Paddon-Jones89) report habitual protein intakes. To our knowledge, only two studies have investigated the acute effects of EAA-based nutritional supplements, containing an optimum ratio of EAA and leucine, on postprandial rates of MPS(Reference Phillips, Chevalier and Leidy50,Reference Katsanos, Kobayashi and Sheffield-Moore95) , appetite and dietary protein intake in concurrent(Reference Ispoglou, Deighton and King93) or subsequent meals(Reference Butterworth, Lees and Harlow62,Reference Ispoglou, Deighton and King93) . The findings demonstrated that EAA-based supplementation may be an effective strategy to facilitate an increased protein intake without compromising intakes of other macronutrients and ultimately energy intake. Ingestion of a gel-based EAA (7⋅5 g EAA) 1 h(Reference Butterworth, Lees and Harlow62,Reference Ispoglou, Deighton and King93) or immediately(Reference Ispoglou, Deighton and King93) before an ad lib breakfast meal significantly increased both protein and energy intakes at the subsequent meal compared to a control condition. Moreover, appetite ratings were not significantly suppressed(Reference Butterworth, Lees and Harlow62,Reference Ispoglou, Deighton and King93) compared to the control condition, while this was not the case for a 15 g EAA-matched whey protein isolate condition(Reference Butterworth, Lees and Harlow62). Energy intake was also increased in the EAA condition compared to the whey protein isolate(Reference Butterworth, Lees and Harlow62), which resulted in an increase of protein intake at the ad lib meal but at the expense of energy intake. The postprandial increase in peptide YY in the whey protein condition offers a potential explanation for the suppression of appetite and ultimately reduced energy intake. This observation may be attributed to a greater increase in amino acid concentration in the gastrointestinal tract(Reference Moran and Dailey96) compared to the EAA condition, which did not contain non-EAA.
Previous research demonstrates that test meal palatability influences food intake to a greater extent when it is followed by disguised high-energy preloads rather than low energy preloads(Reference Yeomans, Lee and Gray97). Other studies(Reference McCrickerd and Forde98) have also provided insights into the influence of sensory inputs, including palatability, on food intake control. It follows that high-energy supplements administered in close proximity or alongside meals should be palatable to allow for additional food consumption and avoid inadvertent energy redistribution. Compliance to any long-term nutritional supplementation regimen is also critical and will be discussed briefly in the section ‘Effects of essential amino acid supplementation alone or in combination with resistance exercise on muscle mass, strength and function’. Ispoglou et al.(Reference Ispoglou, Deighton and King93) considered practical aspects of palatability following different delivery methods of EAA (i.e. a gel or a bar). Participants in their study(Reference Ispoglou, Deighton and King93) rated both EAA containing supplements (i.e. 7⋅5 g EAA) as palatable, suggesting that such nutritional prototypes may be adopted long term by older adults who wish to increase their protein intake without reducing food consumption(Reference Yeomans, Lee and Gray97,Reference Johnson and Wardle99) . Readily administered forms of supplements (i.e. in gel format) are also critical for enhancing protein intakes in older adults with protein-energy malnutrition, which appears to exacerbate the rates of dysphagia with ageing(Reference Hudson, Daubert and Mills42). These findings suggest that palatable forms of EAA supplements may be an efficacious mode of ingestion for enhancing protein intake in older adults with suboptimal protein intakes, a need to maintain or increase protein intake, difficulties with chewing and dysphagia(Reference Hudson, Daubert and Mills42) and/or suppression of taste and smell(Reference Landi, Calvani and Tosato43).
A paucity of studies have investigated the chronic effect of EAA supplementation in augmenting protein intake, and inconsistent approaches have been adopted in relation to the reporting of habitual dietary intakes and habits(Reference Ferrando, Paddon-Jones and Hays88,Reference Rondanelli, Opizzi and Antoniello91,Reference Ispoglou, White and Preston94) . For example, in a 3-month EAA intervention in older obese women, participants were instructed to maintain dietary habits, but dietary intakes were not reported or monitored(Reference Dillon, Sheffield-Moore and Paddon-Jones89). Conversely, studies involving EAA supplementation, such as a 10-d bed rest intervention in healthy older adults with strict dietary controls(Reference Ferrando, Paddon-Jones and Hays88), precludes meaningful conclusions being drawn regarding the efficacy of EAA since the methodological approaches followed in controlled laboratory studies cannot always be applied to real-world settings. It is also not clear if converting nitrogen or amino acid content to protein content is consistently applied. For example, Rondanelli et al.(Reference Rondanelli, Opizzi and Antoniello91) reported that the daily protein intake of older institutionalised adults >75 years was not increased with EAA supplementation when accounting for the nitrogen content from EAA (i.e. 8 g daily), despite improvements in nutritional status including a small but non-significant increase in energy intake. Nevertheless, it is unclear how nitrogen content was calculated. It would be judicious to convert nitrogen to protein content whereby this methodological step would have highlighted a substantial increase in the theoretical protein intake (i.e. using a default factor of 5⋅6, 1 g nitrogen would require intake of 5⋅6 g protein)(Reference Mariotti, Tomé and Mirand100). Adding supplemental EAA to total daily protein content(Reference Rondanelli, Opizzi and Antoniello91) is also an inherently flawed approach given that twice the amount of a high-quality protein would be required to supply a given amount of EAA(Reference Hulmi, Lockwood and Stout101). Hence, it could be speculated that daily protein intake as assessed at the end of this 8-week, dietary intervention(Reference Rondanelli, Opizzi and Antoniello91) was probably underestimated if neither nitrogen to protein nor total EAA to protein conversion was implemented. Similarly, daily supplementation with approximately 15 g EAA alongside two main meals for a period of 12 weeks(Reference Ispoglou, White and Preston94) did not alter habitual energy intakes of healthy older adults. However, accounting for the EAA content in this well-nourished group of older adults, who exceeded recommendations for the current RDA, would have resulted in a daily protein intake closer to 1⋅5 g/kg/d(Reference Hulmi, Lockwood and Stout101). These studies did not report per meal protein intakes, hence further research is warranted to explore whether concurrent or subsequent mealtime intakes, following EAA supplementation, are impacted over the long term. In summary, the few studies that have investigated the efficacy of EAA to augment protein intake provide preliminary evidence that EAA supplementation does not reduce habitual daily dietary intakes, whereas the increase in daily protein intake is due to the supplement administered. It is currently not known how daily EAA supplementation may affect per meal dietary intakes, since most studies administer supplements between meals, while addressing suboptimal per meal protein intakes is likely to address daily protein deficiencies and ultimately augment skeletal muscle responses and adaptations. Since attrition to a nutritional EAA intervention involving capsules may be primarily attributed to palatability and acceptability issues(Reference Ispoglou, White and Preston94), special consideration should be given regarding administration methods (i.e. gels, bars and crystalline EAA) that can safeguard the long-term success of the proposed supplementation regimen.
Effects of essential amino acid supplementation alone or in combination with resistance exercise on muscle mass, strength and function
The acute effects of EAA ingestion on muscle protein turnover have been extensively studied(Reference Park, Church and Azhar85,Reference Katsanos, Kobayashi and Sheffield-Moore95,Reference Volpi, Kobayashi and Sheffield-Moore102–Reference Wilkinson, Bukhari and Phillips106) and provide robust evidence for the role of EAA as key regulators of MPS. However, limited information exists regarding the chronic impact of EAA supplementation in modulating muscle mass, strength and function in older adults. Consistent with this notion, two recent systematic reviews(Reference Tieland, Franssen and Dullemeijer70,Reference Beaudart, Rabenda and Simmons107) summarised the long-term effects of protein-based supplementation, identifying only seven eligible intervention studies using EAA(Reference Ferrando, Paddon-Jones and Hays88,Reference Dillon, Sheffield-Moore and Paddon-Jones89,Reference Rondanelli, Opizzi and Antoniello91,Reference Ispoglou, White and Preston94,Reference Scognamiglio, Piccolotto and Negut108,Reference Kim, Suzuki and Saito109) . As such, these systematic reviews reveal equivocal findings regarding the impact of EAA-based supplementation on muscle mass, strength and function in healthy older adults. Methodological differences inevitably exist between studies with regard to the provision of intact protein sources, individual amino acids or amino acid metabolites that may explain this discord. For instance, two studies administered leucine, which in isolation appears to be ineffective at promoting gains in lean tissue mass(Reference Verhoeven, Vanschoonbeek and Verdijk110,Reference Leenders, Verdijk and van der Hoeven111) . Crucially, the anabolic potential of leucine is only observed in the presence of the other amino acids and in particular the EAA, since a sustained increase in MPS requires the availability of all EAA(Reference Moberg, Apró and Ekblom34,Reference Suryawan, Orellana and Fiorotto112) . Nevertheless, it is vital that EAA mixtures include a relatively high ratio of leucine to achieve maximal stimulation of MPS in older adults(Reference Katsanos, Kobayashi and Sheffield-Moore95).
Most interventions involving EAA only supplementation that report a beneficial effect on muscle mass and functional outcomes have been conducted in patients with obesity and/or sarcopenia(Reference Dillon, Sheffield-Moore and Paddon-Jones89,Reference Rondanelli, Opizzi and Antoniello91,Reference Kim, Suzuki and Saito109,Reference Solerte, Gazzaruso and Bonacasa113,Reference Zhou, Xing and He114) , with a single study conducted in glucose-intolerant older men and women(Reference Borsheim, Bui and Tissier115). This observation emphasises the need for targeted interventions in clinical older populations with underlying sarcopenia and/or obesity. A shared methodological aspect across the majority of these studies is that EAA supplements are administered between meals rather than alongside meals. A major practical implication of this approach may be that older adults with suboptimal per meal protein intakes still fail to meet their per meal protein recommendations, thus the degree and rate of skeletal muscle adaptations may be negatively influenced over the long term. However, in cases where older adults meet per meal protein recommendations, it would be practical to ingest supplements between meals, since rates of MPS would not be increased further due to the ‘muscle-full’ effect(Reference Mitchell, Phillips and Hill87).
In view of the fact that none of the studies discussed here have reported per meal protein intakes, we can only speculate regarding the potential impact on long-term responses and adaptations. Accordingly, in older institutionalised adults, twice-daily supplementation with 4 g EAA (8 g in total, with EAA given as between-meal snacks) was associated with improvements in quality of life, muscle function and diet profile as assessed by the mini nutrition assessment tool(Reference Rondanelli, Opizzi and Antoniello91). Consistent with this finding, whole body lean tissue mass was increased following 18 months of daily supplementation with 16 g EAA (2 × 8 g as between-meal snacks)(Reference Solerte, Gazzaruso and Bonacasa113) in older adults with sarcopenia, whereas insulin sensitivity was improved and insulin-like growth factor 1 was significantly increased. Although MPS was not measured in this study(Reference Solerte, Gazzaruso and Bonacasa113), we speculate that the increase in insulin-like growth factor 1 with EAA supplementation likely increased the stimulation of MPS. Support for this notion also stems from a study by Dillon et al.(Reference Dillon, Sheffield-Moore and Paddon-Jones89), sedentary older women with obesity, who showed that enhanced rates of basal MPS in an EAA-treated group were consistent with increases in lean body mass and protein expression of skeletal muscle insulin-like growth factor 1. The supplementation regimen consisted of a twice-daily (7⋅5 g) dose of EAA (15 g total daily) containing 18⋅6 % l-leucine, and resulted in a marked increase in lean body mass (3⋅9 %) following 3 months of EAA supplementation(Reference Dillon, Sheffield-Moore and Paddon-Jones89), despite the absence of strength gains. In a study focusing on women with sarcopenia(Reference Kim, Suzuki and Saito109), supplementation with 3 g leucine-enriched EAA twice-daily (6 g in total) for a period of 3 months did not result in strength or functional performance improvements, other than walking speed, compared to baseline or a placebo condition. In a similar investigation, with strict dietary controls in place, ingestion of 10 g EAA as snacks twice-daily (20 g in total) over 28 months was shown to increase appendicular skeletal muscle index in patients with sarcopenia and obesity(Reference Zhou, Xing and He114), however no functional outcome measures were reported. Furthermore, Borsheim et al.(Reference Borsheim, Bui and Tissier115), demonstrated that twice-daily supplementation with 11 g EAA including l-arginine (in total 22 g/d) between meals over a 16 week period improved performance and physical function in older adults with glucose intolerance and adequate daily protein intake. In this study, lean body mass was increased by about 1 kg compared to baseline at week 12, but not at week 16 of the intervention. Habitual energy and protein intakes remained unchanged compared to baseline. Nevertheless, the addition of the EAA supplement would have resulted in a daily increase in total protein intake to a level considered optimal for this population. It is unknown whether supplementation enabled participants to meet their per meal protein recommendations, since supplements were ingested between meals rather than alongside meals.
Despite an overall positive impact of supplementation with EAA on muscle mass in populations with obesity, sarcopenia, or compromised health status, positive changes in strength and functional performance are not always evident. Methodological differences (i.e. supplementation between meals), ratio and amount(s) of amino acids used, and lack of exercise interventions alongside EAA supplementation are all potential factors that can explain the divergent findings.
Fewer studies have been conducted in healthy older adults than older adults with sarcopenia or obesity, and these studies tend to utilise more diverse methodological approaches. Nonetheless, preliminary evidence suggests that supplementation with EAA may contribute to functional and strength gains, whereas evidence is weaker with regard to muscle mass increases. For instance, Markofski et al.(Reference Markofski, Jennings and Timmerman116) supplemented healthy older adults with EAA over a 24 week period and failed to detect any increases in total lean body mass, regardless of an increase before and at the end of the intervention in acute EAA-stimulated rates of MPS. Furthermore, neither functional nor strength gains were observed, giving support to the notion that supplementation of EAA alone does not confer any additional benefits. In this study, although daily (15 g) EAA supplementation was strategically timed between meals, it remains unknown whether participants met recommendations for protein intake on a daily and/or per meal basis, since these outcomes were not measured. Conversely, during a 10 d bed rest study in healthy older adults when dietary protein intake was increased to about 1⋅4 g/kg per day (3 × 15 g EAA) during energy balance(Reference Ferrando, Paddon-Jones and Hays88), muscle function was maintained. Nevertheless, muscle mass was reduced despite an increase in protein intake and maintenance of MPS rates, which supports the idea that preservation of muscle function may be due to the maintenance of more functional fibres during inactivity. A study in older adults with no underlying health conditions demonstrated both functional performance gains and an increase in lean tissue mass during 3 months of EAA supplementation while adopting a slightly different methodological approach; participants consumed about 7⋅5 g leucine-enriched (40 %) EAA twice daily (total of 0⋅21 g/kg per day or about 15 g daily)(Reference Ispoglou, White and Preston94). Previous research has demonstrated that physical (in)activity and protein intake may contribute to a blunted response of MPS(Reference Breen, Stokes and Churchward-Venne58,Reference Luiking, Deutz and Memelink117,Reference Burd, Wall and van Loon118) . Thus, it is intuitive that when dietary protein intake is sufficient to maximise MPS, additional supplementation may not confer any muscle anabolic advantage due to a ‘muscle-full’ effect(Reference Mitchell, Phillips and Hill87). In practice, if participants were already receiving adequate high-quality protein with their main meals, nutritional supplementation would not have been of additional benefit, since the required daily protein goal was already reached. Furthermore, in terms of compliance, participants in the study by Ispoglou et al.(Reference Ispoglou, White and Preston94) managed only 75–85 % of the prescribed dose of EAA due to palatability issues and the practicality of ingesting multiple (about 30) capsules on a daily basis. Compliance issues associated with tolerating high numbers of capsules raises important questions regarding long-term compliance to EAA supplementation studies and protein supplementation trials in general, whereby compliance has been shown to be as low as 43 %(Reference Kirk, Mooney and Amirabdollahian119). Strength and functional performance improvements have also been observed in older adults with reduced physical activity levels following a daily supplementation regimen of 3 × 4 g EAA over a 3 month period(Reference Scognamiglio, Piccolotto and Negut108). It is worth acknowledging that benefits associated with EAA supplementation may not be manifested at a skeletal muscle level but rather accrue in other physiological domains (i.e. immunity) and conditions (i.e. energy deficit, catabolic state and injury) that are beyond the scope of this review(Reference Gwin, Church and Wolfe120).
It should be reiterated that sensitivity to the anabolic potential of EAA increases in the presence of exercise, largely through the synergistic influence of mTOR(Reference Sepulveda, Bush and Baar121–Reference Dickinson, Fry and Drummond123). Nevertheless, evidence from acute metabolic studies is equivocal with regard to the efficacy of combining EAA supplementation with resistance exercise to stimulate MPS in older adults. Early research demonstrated a similar, albeit delayed, acute MPS response in older compared with younger adults following the ingestion of 20 g EAA 1 h after cessation of bilateral leg extension exercise (eight sets of ten repetitions; 70 % of one repetition maximum strength)(Reference Drummond, Dreyer and Pennings59). Moreover, the phosphorylation status of key intramyocellular signalling targets in the mTORC1 pathway (i.e. mTOR, S6K1, 4E-BP1 and eEF2) did not differ between age groups. In contrast, other studies have observed a blunting of the mTORC1 pathway(Reference Francaux, Demeulder and Naslain124), when EAA were ingested following resistance exercise. A comparable profile of aminoacidaemia, mTORC1 signalling, and/or MPS has also been documented, irrespective of age(Reference Lees, Wilson and Webb39,Reference Farnfield, Breen and Carey125–Reference Symons, Sheffield-Moore and Mamerow127) . Therefore, it has been suggested that optimising both anabolic stimuli (i.e. EAA/dietary protein and resistance exercise) may effectively counter anabolic resistance in older adults(Reference Fry and Rasmussen128–Reference Marshall, Smeuninx and Morgan131) and augment anabolic responses and adaptations.
Longitudinal studies of combined EAA and resistance exercise interventions in older adults demonstrate inconsistent findings regarding skeletal muscle mass and function. A systematic review of sixteen trials reported beneficial effects of combined EAA or protein supplementation and resistance training on body composition (n 3 studies), strength (n 3 studies) and physical performance (n 2 studies) in older adults(Reference Gade, Pedersen and Beck132). This review concluded that individuals who suffer from sarcopenia and/or frailty could benefit more from combined resistance exercise and EAA or protein supplementation strategies than healthy older adults. However, it should be highlighted that only one study(Reference Kim, Suzuki and Saito109) administered free form EAA, variable methodologies were employed between studies, and no study reported habitual protein intakes which together precludes a definitive conclusion regarding the efficacy of EAA ingestion on muscle mass and function in healthy older adults. Kim et al.(Reference Kim, Suzuki and Saito109) reported increased knee extension strength (9⋅3 %) in community-dwelling older Japanese women with sarcopenia following twice-daily ingestion of a leucine-enriched EAA mixture (6 g daily; 42 % leucine) alongside twice-weekly comprehensive physical training (incorporating resistance-type activities) for 3 months. Leg muscle mass increased in the EAA and exercise group as well as the exercise only group; however, it is noteworthy that EAA supplementation was required to facilitate improvements in knee extension strength. An important population that could benefit from resistance training combined with EAA supplementation is older adults with sarcopenia recovering from injury. In a randomised controlled trial which recruited patients with osteoporotic hip fractures, 2 months of daily EAA (8 g total daily; about 30 % leucine) supplementation in combination with a physical exercise programme including resistance-type activities significantly improved handgrip strength, timed up and go performance, and Iowa Level of Assistance score only in individuals with sarcopenia(Reference Invernizzi, de Sire and D'Andrea133). Therefore, existing evidence indicates that older adults recovering from potentially devastating injuries, such as hip fractures, may improve their functional strength by consuming EAA and engaging in resistance-type exercise activities.
Conclusions and future directions
Ageing per se is associated with sarcopenia, which is exacerbated in older adults with reduced physical activity and malnutrition. The prevalence of sarcopenia is high in institutionalised settings (i.e. care homes), and in older adults with a range of clinical disorders (i.e. obesity, osteoporosis and cancer) or those recovering from disease and injury. Short-term inactivity due to injury or hospitalisation may result in anabolic resistance and further exacerbate rates of sarcopenia in ageing populations. Therefore, optimising energy and protein intakes in older adults is paramount to the successful management and prevention of sarcopenia. Nevertheless, older adults encounter challenges such as dysphagia and suppression of appetite, taste and smell. As such, addressing energy and protein deficiencies by consumption of high-quality proteins on a daily and per meal basis offer evidence-based strategies for managing sarcopenia. However, the satiating properties of protein may create a ‘protein paradox’ whereby an increase in protein intake may be achieved at the expense of other vital nutrients due to partial energy redistribution. This in turn will likely affect the anabolic potential of the main meals (i.e. breakfast, lunch and dinner) and over the long-term compromise skeletal muscle health. We propose that EAA-based supplements enriched with l-leucine may be an alternative strategy to whole protein sources for assisting older adults to meet daily, and more importantly per meal, protein recommendations since the current evidence regarding the long-term impact on muscle mass and function is based primarily on underpowered observational studies. This strategy is particularly relevant during the periods of muscle disuse (i.e. before, during and after hospitalisation) when skeletal muscle becomes less sensitised to anabolic stimuli such as protein. Since resistance exercise enhances the anabolic potential of EAA, exercise interventions should complement, where appropriate, protein-based nutritional interventions to maximise MPS. We specifically emphasise the need to ingest EAA-based supplements alongside meals with suboptimum amounts of protein. However, supplements may be given between meals in cases where older adults receive ample protein with their main meals. Despite the emphasis of this review on maintenance of muscle mass and function, we should not underestimate the role of EAA on positively influencing other aspects of health, such as metabolic health and immunity. In this regard, future research is warranted to explore the feasibility and efficacy of ingesting EAA on addressing per meal and daily protein recommendations in clinical (i.e. prehabilitation and rehabilitation) and institutionalised settings, where rates of sarcopenia, frailty, undernutrition and malnutrition are highly prevalent.
Acknowledgements
We wish to express our sincere thanks to the Nutrition Society for the invitation to contribute to the Proceedings of the Nutrition Society following the Nutrition Society Live 2020 event.
Financial Support
None.
Conflict of Interest
A published European Patent Application (No. EP14734541⋅7, status pending) by one of the authors (T. I.); the associated study was not financially supported by any external commercial organisation.
Authorship
The authors had joint responsibility for all aspects of preparation of this paper.