Introduction
Adoption of a low-carbohydrate diet (LCD) or ketogenic diet (KD) is gaining popularity as a nutritional therapy for many dysfunctions, including those within the nervous system(Reference Christensen, Damsgaard and Fink-Jensen1–Reference Wlodarek3). Both diets focus on the restriction of dietary carbohydrate. An LCD typically reduces intake to below 130 g per day, with a KD further reducing intake to below 50 g per day to induce nutritional ketosis(Reference Miller, Villamena and Volek4) not necessarily achieved with an LCD.
Ketosis is the elevation of ketones in the blood, with a therapeutic KD generally in the range of 0·5–3·0 mmol/l from a reduction in carbohydrate to <50 g per day(Reference Phinney, Horton and Sims5). The production of ketone bodies from fat oxidation can influence neurons in several ways: as an alternate fuel source with potential efficiency gains and reduced oxidative stress compared with glucose metabolism, as a regulator of neurotransmitter production and clearance, as a signalling molecule promoting neuroprotection, and as an epigenetic modulator to up-regulate gene expression of antioxidant, mitochondrial and anti-inflammatory functions(Reference Miller, Villamena and Volek4,Reference Fedorovich, Voronina and Waseem6,Reference Field, Field, Pourkazemi and Rooney7) . As such, ketones have a dual action, both as a fuel source assisting with bioenergetic stability and a signalling molecule regulating many pathways within the nervous system.
An LCD allows for up to 130 g of carbohydrate per day but does not necessarily produce the same level of fat oxidation and may not result in blood ketone elevation. Despite this, smaller reductions in carbohydrate intake still produce favourable metabolic consequences. These include reducing high blood glucose levels that can sensitise sensory neurons and stimulate pro-inflammatory pathways via receptors and channels on the neuron and microglia(Reference Ghosh, Castillo, Frias and Swanson8–Reference Shen, Kapfhamer and Minnella11). Overall, whether the mechanism is glucose reduction, ketone formation or a synergistic relationship between them, the impact of an LCD or a KD on the nervous system is potentially favourable.
Chronic pain is characterised by increased sensitivity within the nervous system and an inflammatory profile(Reference Gerdle, Bäckryd, Falkenberg, Lundström and Ghafouri12,Reference Albrecht, Ahmed and Kettner13) and as such may respond to an LCD or a KD. There is limited research on how low-carbohydrate nutrition influences neurobiology in this population. We have recently reported relevant pre-clinical research which identified 170 animal model studies using a ketogenic diet to produce changes within the nervous system(Reference Field, Field, Pourkazemi and Rooney7). These studies investigated the mechanisms reported to occur with nutritional ketosis and how these alter nervous system function, with fourteen broad mechanistic themes emerging. These included: (a) metabolic, energetic and biochemical changes, (b) restoration of cortical excitability to homoeostatic levels, (c) gene regulation, (d) improved mitochondrial function and number, (e) reduced neuroinflammation, (f) adaptive neuroplasticity, (g) neurotransmitter and synaptic regulation, (h) reduced oxidative stress and (i) activation of signalling pathways. We have also more recently identified a significant and clinically meaningful reduction in reported pain of patients undergoing a whole-food ketogenic diet intervention in a pilot clinical trial(Reference Field, Pourkazemi and Rooney14).
We lacked the capacity to investigate potential neurological mechanisms in that pilot and as such conducted this scoping review. The primary aim of this scoping review was to identify any clinical trials that report changes in adult nervous system function and report outcomes that may be relevant to drivers of chronic pain. The secondary aim was to more broadly identify clinical trials across any research area that report changes in inflammation in response to an LCD or a KD. As such, this scoping review is a more comprehensive approach than other recent neurological reviews that limited their focus to particular diseases or focused on paediatric populations(Reference Christensen, Damsgaard and Fink-Jensen1,Reference Kraeuter, Phillips and Sarnyai15–Reference Liu, Yang and Wang17) . It also considers LCD/KD research focused on other body systems or populations (such as metabolic studies or athletes) that report on how the diet influences inflammatory biomarkers.
Methods
Protocol
The primary research question for this review was: ‘How does an LCD or a KD influence the human adult nervous system?’, with the secondary research question being ‘Does an LCD or a KD influence inflammatory processes?’. Relevant items from the scoping review protocol and PRISMA-ScR checklist from the Joanna Briggs Institute(Reference Peters, Godfrey, McInerney, Aromataris and Munn18,Reference Tricco, Lillie and Zarin19) were used as the framework. Studies were reviewed in three phases. Phase one captured all publications reporting a human ketogenic diet that met the criteria for daily carbohydrate intake, research type and diet duration. Phase two sorted the retrieved papers into either research reporting adult neurological outcomes for the primary analysis or allocated the remaining studies into categories based on reported research focus. Research papers on paediatric populations that met the eligibility criteria were also grouped to allow for simple quantification but not further analysed. Phase three re-examined all adult papers from phase two for studies reporting common inflammatory biomarkers. The purpose of this broad approach was to avoid missing studies with a different research focus that also reported a neurological or inflammatory outcome, to establish the breadth of research in the neurological area within the context of all ketogenic diet research, and to quantify the extent of ketogenic research in human adult populations.
Eligibility criteria
For the first screen, studies were included if they met the following criteria: (a) human studies that reported a low-carbohydrate diet (LCD) (≤130 g per day and/or <26% of total energy intake (TEI)) or ketogenic diet (KD) (≤50 g per day and/or <15% of total energy intake)(Reference Volek, Phinney and Krauss20), (b) the LCD/KD was an intervention, (c) the diet lasted ≥14 days to allow for adaptation to the new diet and (d) the study design was prospective (observational prospective cohorts where the KD/LCD was a treatment, or experimental, longitudinal pre–post intervention trials including randomised controlled trials). Full texts were reviewed if the abstract did not contain this information. Studies were excluded if they: (a) were single case reports, (b) were retrospective reviews, (c) were cross-sectional or associational studies, (d) used exogenous ketones rather than diet, (e) were reviews, commentaries, letters or conference papers, or (f) were not in English where the required information was not in the abstract (Fig. 1).
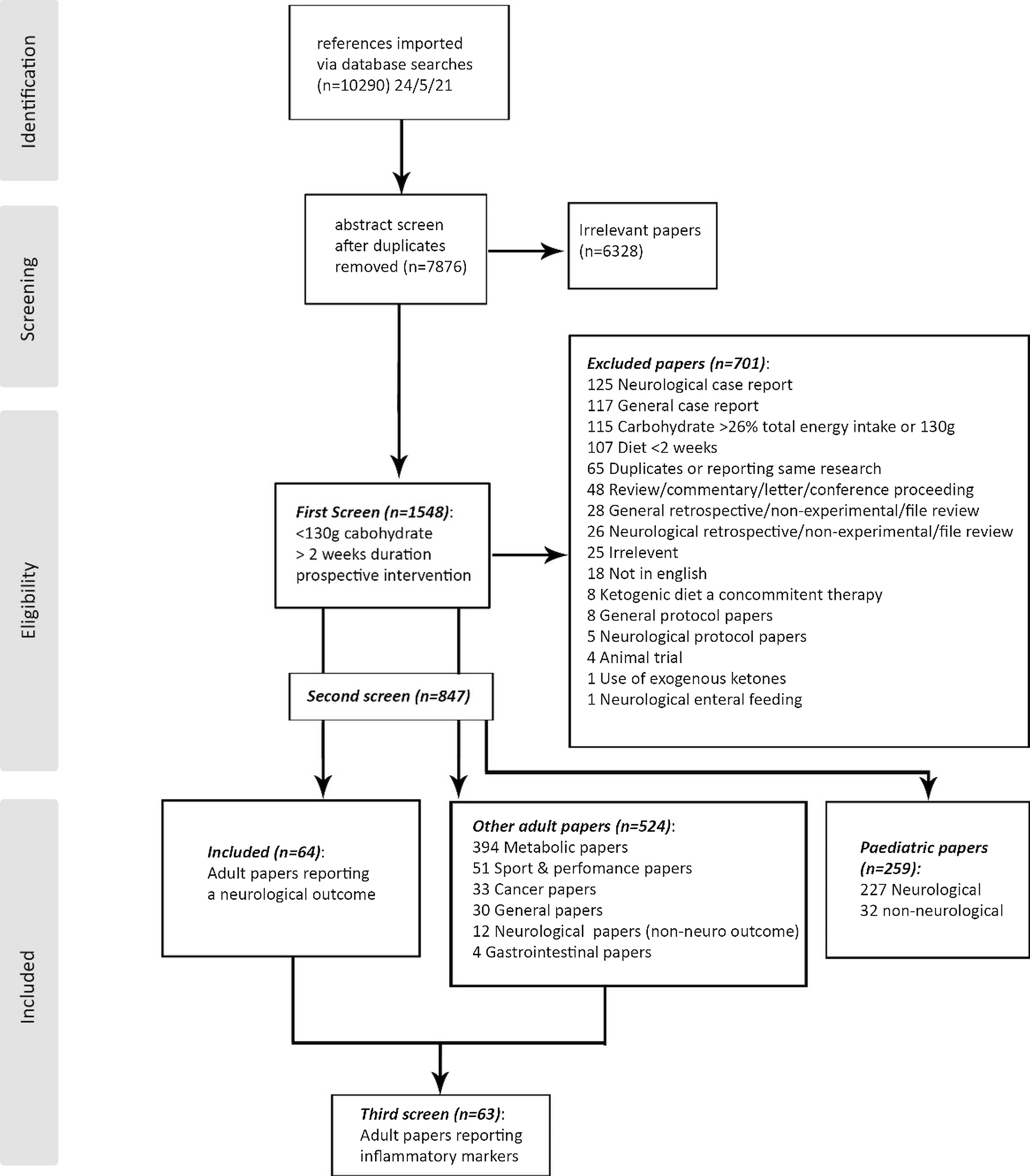
Fig. 1. Inclusion/Exclusion flow chart.
For the second screen, studies were then sorted into three groups: (a) any research on adult populations reporting objective outcomes related to nervous system function, pain, cognitive functioning or psychological outcomes (including mood, anxiety, depression and cognitive processing, but not food cravings or hunger); (b) other adult studies which were categorised by research area: metabolic, cancer, sports/performance, general, gastrointestinal or neurological studies with non-neurological outcomes (for quantification, but did not have data extracted); or (c) paediatric papers (for quantification, but did not have data extracted). Many studies evaluate the effect of a low-carbohydrate or ketogenic diet on child and adolescent development. As this was not the focus of this study, paediatric studies were not included for data extraction to eliminate this confounding variable. We do, however, report the number of studies retrieved.
The third screen searched the two adult groups for any research that reported changes in common biomarkers associated with increased inflammation, including reactive oxygen species (ROS), oxidative stress, C-reactive protein (CRP), tumour necrosis factor alpha (TNF-α), leptin, interleukin (IL)1, 6 or 8, or decreased inflammation (adiponectin, fibroblast growth factor 21 (FGF-21), IL10). A concise summary of the PICOS criteria can be found in Table 1.
Table 1. PICOS criteria for inclusion and exclusion of studies

Information sources and search strategy
An electronic database search including Medline, EMBASE, Cochrane Library for controlled trials, AMED via OVID, CINAHL via EBSCO, Web of Science and PubMed was carried out on 24 May 2021 and included publication dates from database inception to search date. The search targeted any reference to an LCD or a KD intervention using the terms ‘ketogenic’, ‘Atkins’, ‘carbohydrate-restricted diet’ or ‘low-carbohydrate diet’. Additional searches included Google Scholar to check identified articles ‘cited by’ and ‘related articles’ links, and reference checks on identified articles with subsequent hand search for these and inclusion if they met the criteria. Retrieved references were downloaded into EndNote reference management software (Endnote X7·7·1, Thomson Reuters 2016) and then imported using Covidence systematic review software (Veritas Health Innovation, Melbourne, Australia).
Study selection and screening
Duplicates were removed, then titles and abstracts were assessed against the eligibility criteria in Covidence by two reviewers independently (R.F. and T.F.). Full texts of identified studies were then screened and categorised by RF. Studies combining paediatric and adult subjects were included if the data identified the adult results of interest separately or if the mean/median age was ≥18 years. Studies included in the second phase were imported into Endnote with their full reference and abstract. Then the inflammatory biomarker search terms were used to extract any study with these terms in any reference field.
Data items
The primary outcome of interest was changes in adult nervous system function (such as excitability, seizure frequency/duration/magnitude, nerve conduction, neuroinflammation, cognitive function or psychological changes). Additional data extracted included: author, study title, year of study, trial type, diet description, enrolment/completion numbers for the LCD/KD, comparator diet if applicable, age of participants and weight loss. These data items were repeated for inflammatory biomarkers.
Data charting process and synthesis of results
An excel sheet was used to compile extracted data items and generate a table of primary outcomes reported. Primary outcomes were reviewed and summarised by R.F. on the basis of whether the LCD/KD produced positive, neutral or negative outcomes, and whether the comparator diet also had significant positive outcomes.
Results
A systematic database search on 24 May 2021 retrieved 7876 publications after the removal of duplicates. Title and abstract screen extracted 1548 papers that were potentially eligible, with full text review excluding 701. The included studies were sorted into publications reporting a neurological outcome (n = 64), other adult research with a different focus (n = 524: metabolic n = 394, sport/performance n = 51, cancer n = 33, general n = 30, neurological with non-neuro outcomes n = 12, or gastrointestinal n = 4), or paediatric papers (paediatric neurological n = 227, other n = 32). A re-screen of all included papers excluding the paediatric populations (n = 588) for the key inflammatory terms retrieved sixty-three studies reporting on inflammatory outcomes (Fig. 1).
Characteristics of included studies
Neurological outcomes
The primary outcome of interest was results pertaining to adult nervous system function. Of the sixty-four publications reporting neurological outcomes (Table 2), twenty-two provided measures on cortical excitability (nineteen epilepsy studies(Reference Carrette, Vonck and de Herdt21–Reference Zare, Okhovat and Esmaillzadeh39), one narcolepsy(Reference Husain, Yancy, Carwile, Miller and Westman40), one glucose transporter-1 deficiency syndrome(Reference Leen, Mewasingh and Verbeek41) and one general physiology(Reference Cantello, Varrasi and Tarletti42)). Additional categories included fifteen psychological publications (eleven mood and/or cognition(Reference Brinkworth, Buckley, Noakes, Clifton and Wilson43–Reference Harvey, Schofield, Zinn and Thornley55), one schizophrenia(Reference Pacheco, Easterling and Pryer56) and one orexinergic system(Reference Valenzano, Polito and Trimigno57)), twelve neurodegenerative disease publications (three Alzheimer’s disease(Reference Neth, Mintz and Whitlow58–Reference Taylor, Sullivan, Mahnken, Burns and Swerdlow60), three multiple sclerosis(Reference Bock, Karber and Kuhn61–Reference Lee, Titcomb and Bisht63), three cognitive impairment(Reference Brandt, Buchholz and Henry-Barron64–Reference Morrison, Fazeli and Gower66) and three Parkinson’s disease(Reference Koyuncu, Fidan, Toktas, Binay and Celik67–Reference VanItallie, Nonas and Di Rocco69)), seven migraine publications(Reference Barborka70–Reference Di Lorenzo, Pinto and Ienca76), two musculoskeletal studies (one hip osteoarthritis/pain(Reference Hall, Spiers and Knox77) and one knee osteoarthritis/pain(Reference Strath, Lukens and Jones78)), two autonomic nervous system studies (one sympathetic activation(Reference Fagerberg, Andersson and Nilsson79) and one heart rate variability(Reference Maunder, Dulson and Shaw80)), two nervous system bioenergetics papers (one cerebral glucose uptake(Reference Lobley, Johnstone and Fyfe81) and one cerebral blood flow(Reference Holsen, Hoge and Lennerz82)), one spinal cord injury paper(Reference Yarar-Fisher, Kulkarni and Li83) and one traumatic brain injury paper(Reference Rippee, Chen and Taylor84). Half of the included studies were randomised controlled trials (RCT) (n = 32), thirty were prospective single or two-arm studies, and two were case series(Reference Leen, Mewasingh and Verbeek41,Reference Barborka70) . Time on the diet ranged between 2 weeks(Reference Cantello, Varrasi and Tarletti42,Reference Pacheco, Easterling and Pryer56) and 112 weeks(Reference Cervenka, Henry, Felton, Patton and Kossoff23) (mean 18 weeks, standard deviation (SD) ± 19), with fifty-seven studies (90%) considered KD <50 g carbohydrate per day, four considered LCD(Reference Lee, Titcomb and Bisht63,Reference Hall, Spiers and Knox77,Reference Fagerberg, Andersson and Nilsson79,Reference Holsen, Hoge and Lennerz82) (between 50 g and 130 g), two unclear(Reference Pacheco, Easterling and Pryer56,Reference Barborka70) and one including LCD and KD(Reference Harvey, Schofield, Zinn and Thornley55). Overall, a total of 1729 participants commenced an LCD or a KD that reported a neurological outcome. Number of participants in trials ranged between 2 and 101, with an average of 26 ± 20. Adherence to the diet was reported between 22% and 100% (mean 79 ± 21%).
Table 2. Reported neurological outcomes from human studies utilising low-carbohydrate (LCD) or ketogenic (KD) diets n = 64

AED, anti-epileptic drugs; AHA, American Heart Association; ALZ, Alzheimer’s disease; BL, baseline; carb, carbohydrate; CR, calorie restriction; CVD, cardiovascular disease; DG, dietary guidelines; EEG, electroencephalogram; GLUT1DS, glucose transporter-1 deficiency syndrome; HC, high carbohydrate; kcal, kilocalorie; KD, ketogenic diet <50 g carbohydrate per day or 15% TEI; LCD, low-carbohydrate diet 50–130 g carbohydrate per day or 16–26% TEI; LCKD, low calorie ketogenic diet (typically between 1000 and 1800 kcal per day); MCI, mild cognitive impairment; MED, Mediterranean diet; MOH, medication overuse headache; MS, multiple sclerosis; N, No; NR, not reported; NS, not significant; NSPME, North Sea Progressive Myoclonus Epilepsy; NSSQ, Narcolepsy Symptom Status Questionnaire; PD, Parkinson’s disease; RCT, randomised controlled trial; rMSSD, root mean square of the sum of successive difference in R-R intervals; T2D, type 2 diabetes; TEI, total energy intake; TMS, transcranial; Uni, university; VLCKD, very-low-calorie ketogenic diet (typically below 1000 kcal per day); VLCnKD, very-low-calorie NON ketogenic diet; VLED, very-low-energy diet.
** Carbohydrate presented as either (a) ratio of fat: protein + carbohydrate, (b) grams per day or (c) a daily percentage.
# Comparator diet also showed significant positive outcome.
Most studies (n = 53, 83%) reported favourable neurological outcomes attributable to carbohydrate restriction. These included: reduction in seizure frequency, improved psychological mood states with reduced negative affect, better cognitive and memory functions, reduced narcoleptic sleepiness, reduced fatigue, depression and rate of multiple sclerosis relapse, reduced structural changes and improved cognitive function in Alzheimer’s disease, improved motor function in Parkinson’s disease, reduced migraine frequency and severity, reduced pain in osteoarthritis, reduced sympathetic nervous system activation, improved heart rate variability and improved recovery following spinal cord injury. Ten papers reported neutral results(Reference van Egmond, Weijenberg and van Rijn38,Reference D’Anci, Watts, Kanarek and Taylor46,Reference Iacovides, Goble, Paterson and Meiring48,Reference Makris, Darcey and Rosenbaum49,Reference Wing, Vazquez and Ryan52,Reference Rosen, Hunt, Sims and Bogardus53,Reference Phillips, Deprez and Mortimer59,Reference Lee, Titcomb and Bisht63,Reference Lobley, Johnstone and Fyfe81,Reference Rippee, Chen and Taylor84) and one trial reported a negative effect on mood(Reference Butki, Baumstark and Driver45). Three papers reported also similar or better improvements for the comparator diet(Reference Brinkworth, Buckley, Noakes, Clifton and Wilson43,Reference Brinkworth, Luscombe-Marsh and Thompson44,Reference Halyburton, Brinkworth and Wilson47) .
Energy intake varied between studies; most reported ad libitum food intake or intake formulated for weight maintenance (n = 43), eight had mild-to-moderate calorie restriction(Reference Klein, Janousek, Barber and Weissberger27,Reference Smith, Politzer, Macgarvie, McAndrews and Del Campo37,Reference Brinkworth, Buckley, Noakes, Clifton and Wilson43,Reference Brinkworth, Luscombe-Marsh and Thompson44,Reference Halyburton, Brinkworth and Wilson47,Reference Phillips, Murtagh, Gilbertson, Asztely and Lynch68,Reference Fagerberg, Andersson and Nilsson79,Reference Lobley, Johnstone and Fyfe81) , six were very low-calorie (<800 kcal per day)(Reference Wing, Vazquez and Ryan52,Reference Rosen, Hunt, Sims and Bogardus53,Reference Valenzano, Polito and Trimigno57,Reference Di Lorenzo, Coppola and Sirianni72,Reference Di Lorenzo, Pinto and Ienca76,Reference Hall, Spiers and Knox77) , three had combined protocols(Reference Mohorko, Cernelic-Bizjak and Poklar-Vatovec51,Reference Bongiovanni, Benedetto and Corvisieri71,Reference Di Lorenzo, Coppola and Bracaglia73) and did not specify energy intake(Reference Pacheco, Easterling and Pryer56,Reference Koyuncu, Fidan, Toktas, Binay and Celik67,Reference Barborka70) . Weight loss pre–post intervention was common (n = 45), but not always significant. Five studies reported no weight loss(Reference Kishk, Yousof and Ebraheim26,Reference McDonald, Henry-Barron and Felton32,Reference Sirven, Whedon and Caplan36,Reference van Egmond, Weijenberg and van Rijn38,Reference Iacovides, Goble, Paterson and Meiring48) , and nine did not report weight changes(Reference Green, Nguyen, Kaalund-Hansen, Rajakulendran and Murphy25,Reference Butki, Baumstark and Driver45,Reference Rosen, Hunt, Sims and Bogardus53,Reference Pacheco, Easterling and Pryer56,Reference Lee, Titcomb and Bisht63,Reference Brandt, Buchholz and Henry-Barron64,Reference Koyuncu, Fidan, Toktas, Binay and Celik67,Reference Barborka70,Reference Yarar-Fisher, Kulkarni and Li83) .
Inflammatory markers
The secondary outcome of interest was measures related to inflammation. A second screen of studies meeting the criteria retrieved sixty-three studies reporting on inflammatory biomarkers (Table 3). These were mostly metabolic studies (n = 52) investigating chronic lifestyle diseases such as diabetes, obesity and heart disease(Reference Valenzano, Polito and Trimigno57,Reference Al-Sarraj, Saadi, Calle, Volek and Fernandez85–Reference Waldman, Smith and Lamberth135) . The remaining studies included three general population(Reference Mohorko, Cernelic-Bizjak and Poklar-Vatovec51,Reference Cipryan, Maffetone, Plews and Laursen136,Reference Cipryan, Dostal, Plews, Hofmann and Laursen137) , two cancer(Reference Hagihara, Kajimoto and Osaga138,Reference Khodabakhshi, Akbari and Mirzaei139) , two neurodegenerative disease(Reference Bock, Karber and Kuhn61,Reference Brenton, Banwell and Bergqvist62) , one auto-immune(Reference Schmidt, Christensen and Serifovski140), one musculoskeletal(Reference Strath, Lukens and Jones78), one sport/performance(Reference Paoli, Cenci and Pompei141) and one spinal cord injury(Reference Yarar-Fisher, Kulkarni and Li83). There was a large proportion of RCTs (n = 45), seventeen prospective studies and one case series(Reference Hagihara, Kajimoto and Osaga138). Time on the diet ranged from 2 weeks(Reference Bock, Karber and Kuhn61,Reference Boden, Sargrad, Homko, Mozzoli and Stein89) to 104 weeks(Reference Athinarayanan, Adams and Hallberg86,Reference Bluher, Rudich and Kloting88) (mean 16 ± 20 weeks) with fifty-three studies considered a KD, five mixed models(Reference Bluher, Rudich and Kloting88,Reference Buscemi, Verga, Tranchina, Cottone and Cerasola92,Reference Davis, Crandall and Gajavelli96,Reference Miller, Volpe, Coleman-Kelly, Gwazdauskas and Nickols-Richardson113,Reference O’Brien, Brehm and Seeley117) and five LCDs(Reference Jonasson, Guldbrand, Lundberg and Nystrom107,Reference Kerksick, Wismann-Bunn and Fogt109,Reference Zadeh, Kargarfard, Marandi and Habibi134,Reference Waldman, Smith and Lamberth135,Reference Schmidt, Christensen and Serifovski140) . The most reported inflammatory biomarker was CRP, followed by TNFα, then various ILs. Studies focused on weight loss commonly reported leptin and adiponectin.
Table 3. Reported inflammatory biomarkers from human studies utilising low-carbohydrate (LCD) or ketogenic (KD) diets, n = 63

ADA, American Diabetes Association diet; AI, anti-inflammatory; ALOX5, arachidonate 5-lipoxygenase; BDNF, brain-derived neurotrophic factor; BL, baseline; BMI, body mass index; COX, cyclooxygenase; CRP, C-reactive protein; CVD, cardiovascular disease; DG, dietary guidelines; DHA, docosahexaenoic acid; EE, energy expenditure; F, female; FGF21, fibroblast growth factor 21; HC, high-carbohydrate; hsCRP, high sensitivity C-reactive protein; HF, high fat; HUF, high unsaturated fat; ICAM-1, intracellular cellular adhesion molecule-1; IFN-γ, interferon gamma; IL, interleukin; kcal, kilocalorie; KD, ketogenic diet; kJ, kilojoule; LF, low-fat; M, male; MC, moderate-carbohydrate; MCP-1, monocyte chemotactic protein-1; MCT, medium-chain triacylglycerol; MD, Mediterranean diet; MetS, metabolic syndrome; NAFLD, non-alcoholic fatty liver disease; NR, not reported; O3, omega-3; PAI-1, plasminogen-activator inhibitor-1; ROS, reactive oxygen species; SF, saturated fat; T1D, type 1 diabetes; T2D, type 2 diabetes; TBARS, thiobarbituric acid reactive substances; TNF-α, tumour necrosis factor alpha; USF, unsaturated fat; VLCKD, very-low-calorie ketogenic diet; VLCnKD, very-low-calorie non-ketogenic diet.
** Carbohydrate presented as either (a) ratio of fat: protein + carbohydrate, (b) grams per day or (c) a daily percentage.
# Comparator diet showed significant positive outcome.
Forty-four studies reported positive outcomes (lowered inflammatory markers) from the diet, with fourteen of these also reporting benefits for the comparator diet(Reference Brinkworth, Noakes, Buckley, Keogh and Clifton91,Reference Dansinger, Gleason, Griffith, Selker and Schaefer95,Reference Gyorkos, Baker and Miutz103,Reference Johnstone, Lobley and Horgan106,Reference Keogh, Brinkworth and Noakes108,Reference Kerksick, Wismann-Bunn and Fogt109,Reference Miller, Volpe, Coleman-Kelly, Gwazdauskas and Nickols-Richardson113,Reference Noakes, Foster and Keogh116,Reference O’Brien, Brehm and Seeley117,Reference Ratliff, Mutungi, Puglisi, Volek and Fernandez122,Reference Sharman and Volek127,Reference Tay, Brinkworth, Noakes, Keogh and Clifton129,Reference Vetter, Wade and Womble131,Reference Zadeh, Kargarfard, Marandi and Habibi134) . Fifteen studies presented equivocal results(Reference Brenton, Banwell and Bergqvist62,Reference Ballard, Quann and Kupchak87,Reference Breukelman, Shaw and Basson90,Reference Buscemi, Verga, Tranchina, Cottone and Cerasola92,Reference Davis, Crandall and Gajavelli96,Reference Forsythe, Phinney and Feinman100,Reference Kong, Sun and Shi110,Reference Merra, Gratteri and De Lorenzo112,Reference Miller, Beach and Sorkin114,Reference Retterstol, Svendsen, Narverud and Holven123,Reference Stoernell, Tangney and Rockway128,Reference Volek, Sharman, Gomez, Scheett and Kraemer132,Reference Waldman, Smith and Lamberth135,Reference Hagihara, Kajimoto and Osaga138,Reference Schmidt, Christensen and Serifovski140) (no significant change in inflammatory markers), two reported mixed results(Reference Mohorko, Cernelic-Bizjak and Poklar-Vatovec51,Reference Rosenbaum, Hall and Guo124) and two studies reported negative results(Reference Johnston, Tjonn and Swan105,Reference Rankin and Turpyn121) (increased inflammatory markers). Total energy intake was similar to the neurological outcomes group; thirty-eight were ad libitum/weight maintenance studies, fifteen had mild-to-moderate calorie restriction(Reference Brinkworth, Noakes, Buckley, Keogh and Clifton91,Reference Buscemi, Verga, Tranchina, Cottone and Cerasola92,Reference Johnston, Tjonn and Swan105–Reference Kerksick, Wismann-Bunn and Fogt109,Reference Kreider, Rasmussen and Kerksick111,Reference Noakes, Foster and Keogh116,Reference Ruth, Port and Shah125,Reference Sharman and Volek127,Reference Tay, Brinkworth, Noakes, Keogh and Clifton129,Reference Volek, Sharman, Gomez, Scheett and Kraemer132,Reference Zadeh, Kargarfard, Marandi and Habibi134) , seven were very low-calorie (<800 kcal per day) (Reference Valenzano, Polito and Trimigno57,Reference Castaldo, Pagano and Grimaldi93,Reference de Luis, Domingo and Izaola97,Reference Gu, Zhao and Huang102,Reference Merra, Gratteri and De Lorenzo112,Reference Monda, Polito and Lovino115,Reference Perticone, Maio and Sciacqua120) and three used mixed models(Reference Mohorko, Cernelic-Bizjak and Poklar-Vatovec51,Reference Castaldo, Rastrelli and Galdo94,Reference Gomez-Arbelaez, Crujeiras and Castro101) . Fifty-seven studies reported weight loss, two reported no weight loss(Reference Ebbeling, Feldman and Klein98,Reference Miller, Beach and Sorkin114) and four did not report a finding(Reference Yarar-Fisher, Kulkarni and Li83,Reference Breukelman, Shaw and Basson90,Reference Zadeh, Kargarfard, Marandi and Habibi134,Reference Khodabakhshi, Akbari and Mirzaei139) . Overall, a total of 2296 participants commenced an LCD or KD trial that reported an inflammatory outcome. Number of participants in trials ranged between 4 and 262, with an average of 35 ± 38. Adherence to the diet was reported between 38% and 100% (mean 87 ± 15%).
Other findings
There are a substantial number of interventional human KD and LCD trials in other research areas reported in the literature that meet the carbohydrate intake criteria and exceed 2 weeks in duration. In addition to the 61 neurological trials, other publications retrieved in the search included: 393 metabolic, 51 sport/performance, 33 cancer, 30 general, 12 neurological (reporting non-neurological outcomes) and 4 gastrointestinal (Supplementary Appendices 2–7).
Discussion
The primary aim of this scoping review was to review adult human studies that report outcomes related to the nervous system in response to an LCD or a KD. The secondary aim was to review the research that reports on changes in inflammatory biomarkers and evaluate the relevance of these findings to chronic pain. The overall results (83% of studies) suggest a favourable outcome on the nervous system from a reduction in dietary carbohydrates. Most studies utilised a KD below 50 g carbohydrate per day (fifty-eight out of sixty-four focused on neurological outcomes (91%), and fifty-five out of sixty-three focused on inflammatory biomarkers (87%)). The more moderate approach to carbohydrate restriction of the LCD also reported positive outcomes, with three out of four LCDs and both ‘unclear’ diets reporting improved neurological outcomes. Similarly, three out of four LCDs and four out of five mixed protocols also reported favourable reductions in inflammatory biomarkers.
The KD is reported to reduce nervous system excitability through ketone signalling. The actions include: activation of various ion channels (such as ATP-sensitive K+ channels) where ketones reduce excitability, modulation of neurotransmitter levels via the reduction of excitatory glutamate and/or increase in inhibitory GABA (or changes in clearance rates), and improved mitochondrial respiration and number with reduced reactive oxygen species formation(Reference Gano, Patel and Rho142,Reference Murano, Binda and Palestini143) . Most studies focused on cortical excitability (twenty-one of twenty-two publications) and reported improvements in seizure measures. Our recent scoping review of animal research using KDs(Reference Field, Field, Pourkazemi and Rooney7) identified fifty-three studies reporting on cortical/neuronal excitability, with forty-two reporting improvements that shifted nervous system excitability back towards homoeostatic levels. Increased neuronal excitability (sensitisation) is common in chronic pain presentations(Reference Nijs, George and Clauw144–Reference Parker, Lewis, Rice and McNair146) and as such may also respond positively to the mechanisms outlined in the review. Additionally, the use of anti-seizure/anti-epileptic medication (such as pregabalin) is commonly prescribed for neuropathic pain, providing further evidence for common pathways being involved in both chronic pain and seizure disorders(Reference Masino and Ruskin147). Given that poor nutrition potentially triggers mechanisms responsible for driving increased nervous system sensitivity(Reference Nijs, Elma and Yilmaz148), nutritional strategies to address chronic pain are potential treatment options. A diet that potentially reduces inflammation is also an option.
KD has also been reported to reduce inflammatory loading, which is supported by the results from this review. Increased levels of inflammatory cytokines (such as CRP, TNF-α and ILs 1, 6 and 8) have been demonstrated in chronic pain presentations, with a potential link between an increase in low-grade inflammation and the development of persisting pain(Reference Gerdle, Bäckryd, Falkenberg, Lundström and Ghafouri12,Reference Schistad, Stubhaug, Furberg, Engdahl and Nielsen149,Reference Andrade, Cornips and Sommer150) . Within the nervous system, pro-inflammatory mediators can activate the microglia, increasing their number and size, altering morphology and increasing receptor expression. This results in further up-regulation of neuroinflammation leading to maladaptive synaptic plasticity and central sensitisation(Reference Ji, Nackley, Huh, Terrando and Maixner151–Reference Vergne-Salle and Bertin154). In contrast, the presence of ketones and/or the suppression of glycolysis positively influence the microglia, shifting them towards anti-inflammatory phenotypes(Reference Morris, Puri and Maes155). In addition, ketone bodies acting as signalling molecules can mitigate NOD-, LRR- and pyrin domain-containing protein 3 (NLRP3) inflammasome activity in the microglia and central nervous system, and act as a ligand for G-protein receptors becoming class 1 and 2 histone deacetylase (HDAC) inhibitors within the brain up-regulating anti-inflammatory and antioxidant pathways(Reference Fedorovich, Voronina and Waseem6,Reference Morris, Puri and Maes155) . Inducing ketosis via a KD to mitigate inflammatory processes can be supported mechanistically(Reference Field, Field, Pourkazemi and Rooney7,Reference Morris, Puri and Carvalho156) as well as clinically from the human studies retrieved (Table 3).
All seven migraine studies utilised a KD and reported positive outcomes. There are several mechanisms relevant to migraine development that are influenced by a KD. In addition to cortical excitability and inflammation already discussed, an energy deficit from impaired glucose metabolism/transport, mitochondrial dysfunction and oxidative stress are also implicated in migraine as well as other neurodegenerative disorders(Reference Gross, Klement, Schoenen, D’Agostino and Fischer157,Reference Morris, Maes, Berk, Carvalho and Puri158) . In the context of glucose restriction, ketones are supplied to the neuron for fuel from the periphery through the blood–brain barrier, but also by fatty acid oxidation occurring in within the astrocyte(Reference Morris, Maes, Berk, Carvalho and Puri158). Ketones can meet the energy shortfall and provide bioenergetic stability. They also reduce oxidative stress by up-regulating antioxidant defence and improved mitochondrial respiration(Reference Miller, Villamena and Volek4,Reference Puchalska and Crawford159) . Whilst the presence of ketones on a KD provides a plausible mechanistic explanation for pain reduction, the absence of ketones on an LCD does not necessarily remove the potential benefit.
An LCD which does not achieve significant levels of blood ketones may also improve chronic pain through mechanisms related to lower blood glucose levels rather than ketone-mediated actions. Chronic hyperglycaemia results in the production of advanced glycation end products which activate receptors on the neuron, potentially damaging it and promoting inflammation (such as in diabetic neuropathy)(Reference Bestall, Hulse and Blackley10). It also results in hyper-insulinaemia where some cells fail to respond correctly to insulin signalling (insulin resistance) linked to the development of chronic pain such as fibromyalgia(Reference Pappolla, Manchikanti and Candido160). A reduction in glucose also reduces the NADH:NAD+ ratio, which signals a reduction in the transcription of pro-inflammatory genes in the microglia(Reference Shen, Kapfhamer and Minnella11). Interestingly, cross-sectional studies investigating the effect of glucose-lowering medication such as metformin report reduced musculoskeletal pain(Reference Carvalho-e-Silva, Harmer, Ferreira and Ferreira161). Overall, the beneficial outcomes on pain from an LCD or a KD may be a synergistic action of lowered blood glucose and increased blood ketones.
Defining the most beneficial diet for chronic pain has been the target of recent systematic reviews(Reference Field, Pourkazemi, Turton and Rooney162–Reference Elma, Yilmaz and Deliens164); however no clear diet stands out(Reference Field, Pourkazemi, Turton and Rooney162). Only two studies using a low-carbohydrate approach specifically for pain were found during this review(Reference Hall, Spiers and Knox77,Reference Strath, Lukens and Jones78) . Both demonstrated improvements in pain outcomes, one using a KD(Reference Strath, Lukens and Jones78) and the other an LCD(Reference Hall, Spiers and Knox77); however, neither presented ketone levels or measured dietary carbohydrate data. A third study(Reference Field, Pourkazemi and Rooney14) published after the scoping review date also reported significant improvements in pain outcomes using a KD protocol with a significant but small rise in ketone levels and measured daily carbohydrate intake reduced to 70 g per day in the intervention group. The human trials presented support the use of a KD for a reduction in nervous system sensitivity; however, an LCD also reports benefits. It is unclear from the current research what level of carbohydrate restriction is required, whether the effect increases proportionately with carbohydrate reduction, or what diet duration length is required for favourable impacts on nervous system sensitisation. Further research using larger participant numbers is required to help answer these questions which are relevant for clinical application.
A limitation of this review is the confounding variable of weight loss. A large portion of included studies reported significant weight loss, which is also reduces inflammatory cytokines and may be also responsible for positive outcomes. A further limitation was the exclusion of extensive paediatric studies that may have provided further mechanistic evidence (such as Napolitano and colleagues(Reference Napolitano, Longo and Lucignani165) who were the first to show an increase in the antioxidant glutathione in human rather than animal models) (Supplementary Appendix 1). Despite this, an LCD or a KD is supported by mechanistic animal research as well as the human data presented here to provide plausible rationale as to how physiology might be influenced in a way to reduce pain perception.
Conclusion
This scoping review of LCD and KD research identified a large body of prospective adult human dietary intervention trials that reduced carbohydrate intake to below 130 g per day and exceeded 2 weeks in length. From these, there were sixty-four studies reporting neurological outcomes of which 83% showed improvement, and sixty-three studies reporting inflammatory biomarkers of which 71% improved. Both nervous system sensitisation and inflammation occur in chronic pain and as such may be improved by low-carbohydrate nutritional therapy. More clinical trials within this population are required to build on the few human trials that have been done.
Acknowledgements
This research did not receive any specific grant from funding agencies in the public, commercial or not-for-profit sector. The authors declare that there is no conflict of interest regarding the publication of this article.
Supplementary material
To view supplementary material for this article, please visit https://doi.org/10.1017/S0954422422000087