Book contents
- What is Life? On Earth and Beyond
- Additional material
- What is Life? On Earth and Beyond
- Copyright page
- Dedication
- Contents
- Contributors
- Preface
- Introduction
- Science
- 1 Reflections on Origins, Life, and the Origins of Life
- 2 The Search for Another Earth-Like Planet and Life Elsewhere
- 3 The Shape of Life: Morphological Signatures of Ancient Microbial Life in Rocks
- 4 Precellular Evolution and the Origin of Life: Some Notes on Reductionism, Complexity and Historical Contingency
- Philosophy
- Theology
- Conclusion
- Index
- References
3 - The Shape of Life: Morphological Signatures of Ancient Microbial Life in Rocks
from Science
Published online by Cambridge University Press: 08 July 2017
- What is Life? On Earth and Beyond
- Additional material
- What is Life? On Earth and Beyond
- Copyright page
- Dedication
- Contents
- Contributors
- Preface
- Introduction
- Science
- 1 Reflections on Origins, Life, and the Origins of Life
- 2 The Search for Another Earth-Like Planet and Life Elsewhere
- 3 The Shape of Life: Morphological Signatures of Ancient Microbial Life in Rocks
- 4 Precellular Evolution and the Origin of Life: Some Notes on Reductionism, Complexity and Historical Contingency
- Philosophy
- Theology
- Conclusion
- Index
- References
Summary
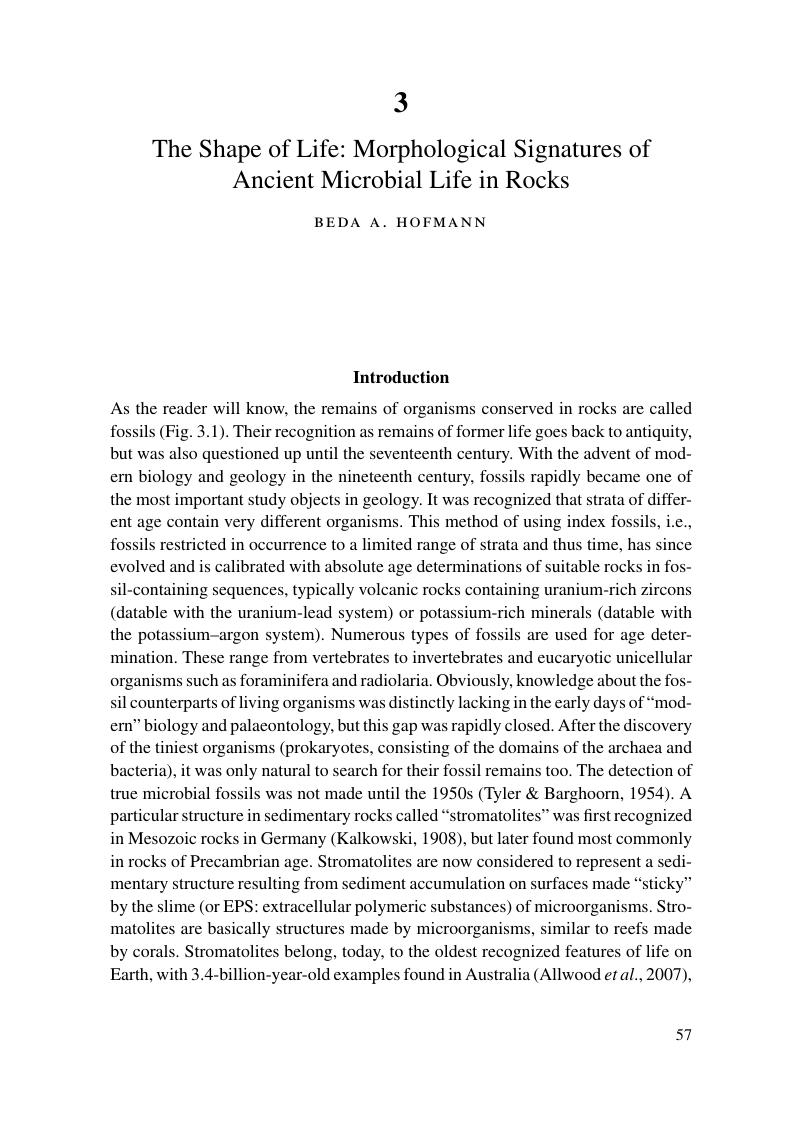
- Type
- Chapter
- Information
- What is Life? On Earth and Beyond , pp. 57 - 74Publisher: Cambridge University PressPrint publication year: 2017