Book contents
- Water Quality Impacts of the Energy-Water Nexus
- Water Quality Impacts of the Energy-Water Nexus
- Copyright page
- Contents
- Acknowledgments
- 1 Introduction
- 2 Quantification of Energy and Water Flows
- 3 The Coal–Water Nexus
- 4 Conventional Crude Oil and Tight Oil–Water Nexus
- 5 Conventional and Unconventional Natural Gas–Water Nexus
- 6 Integration
- References
- Index
- Plate Section (PDF Only)
- References
References
Published online by Cambridge University Press: 03 February 2022
- Water Quality Impacts of the Energy-Water Nexus
- Water Quality Impacts of the Energy-Water Nexus
- Copyright page
- Contents
- Acknowledgments
- 1 Introduction
- 2 Quantification of Energy and Water Flows
- 3 The Coal–Water Nexus
- 4 Conventional Crude Oil and Tight Oil–Water Nexus
- 5 Conventional and Unconventional Natural Gas–Water Nexus
- 6 Integration
- References
- Index
- Plate Section (PDF Only)
- References
Summary
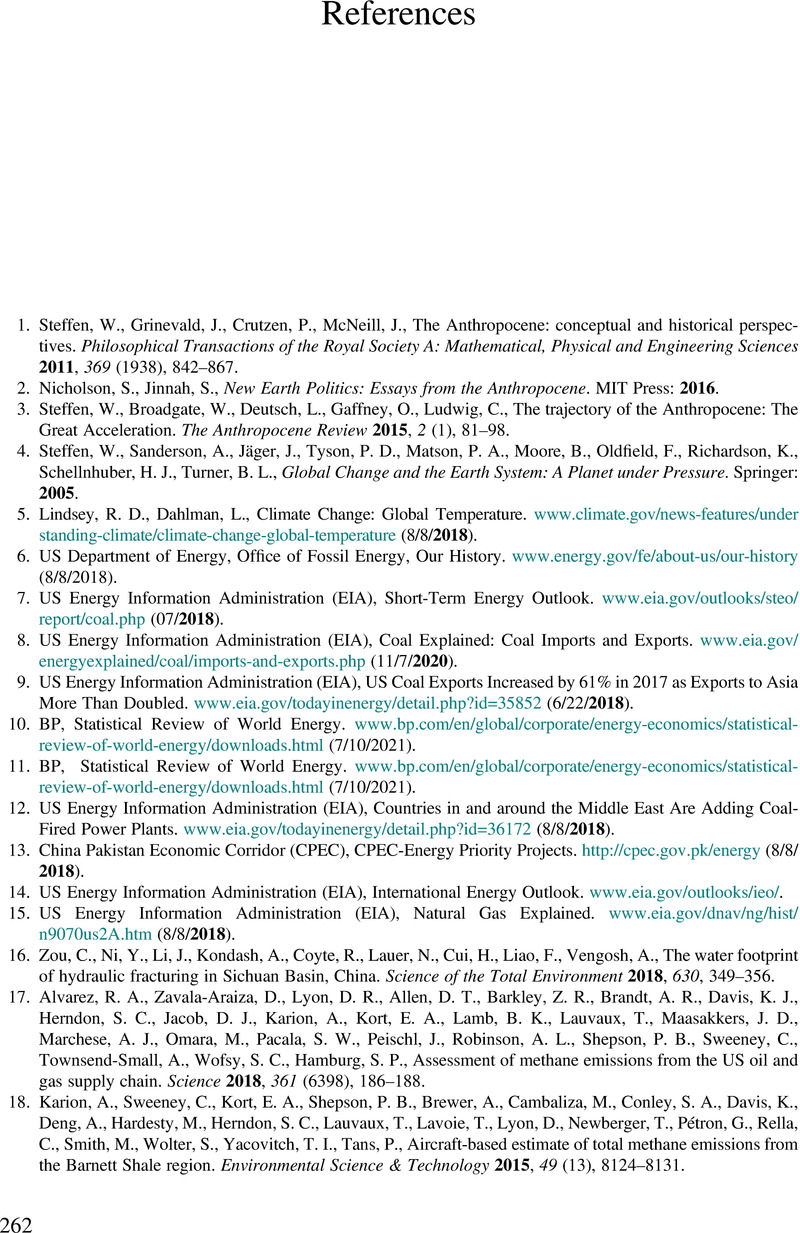
- Type
- Chapter
- Information
- Water Quality Impacts of the Energy-Water Nexus , pp. 262 - 300Publisher: Cambridge University PressPrint publication year: 2022