Book contents
- Volcanism and Global Environmental Change
- Volcanism and Global Environmental Change
- Copyright page
- Table of contents
- List of contributors
- Preface
- Part One Large volume volcanism: origins, features and timing
- 1 Large igneous provinces and explosive basaltic volcanism
- 2 On the nature and consequences of super-eruptions
- 3 Large igneous province locations and their connections with the core–mantle boundary
- 4 High-precision U–Pb geochronology of Phanerozoic large igneous provinces
- 5 Volcanic pulses in the Siberian Traps as inferred from Permo-Triassic geomagnetic secular variations
- Part Two Assessing gas and tephra release in the present day and palaeo-record
- Part Three Modes of volcanically induced global environmental change
- Index
1 - Large igneous provinces and explosive basaltic volcanism
from Part One - Large volume volcanism: origins, features and timing
Published online by Cambridge University Press: 05 February 2015
- Volcanism and Global Environmental Change
- Volcanism and Global Environmental Change
- Copyright page
- Table of contents
- List of contributors
- Preface
- Part One Large volume volcanism: origins, features and timing
- 1 Large igneous provinces and explosive basaltic volcanism
- 2 On the nature and consequences of super-eruptions
- 3 Large igneous province locations and their connections with the core–mantle boundary
- 4 High-precision U–Pb geochronology of Phanerozoic large igneous provinces
- 5 Volcanic pulses in the Siberian Traps as inferred from Permo-Triassic geomagnetic secular variations
- Part Two Assessing gas and tephra release in the present day and palaeo-record
- Part Three Modes of volcanically induced global environmental change
- Index
Summary
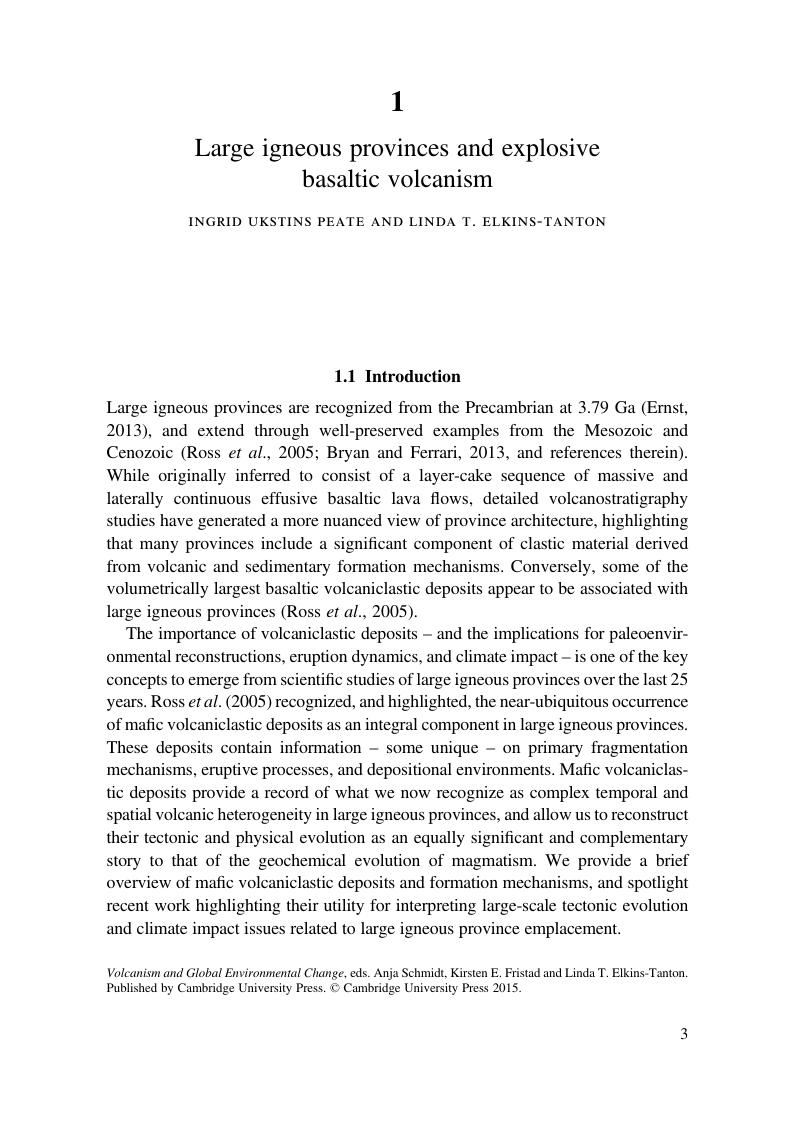
- Type
- Chapter
- Information
- Volcanism and Global Environmental Change , pp. 3 - 15Publisher: Cambridge University PressPrint publication year: 2015
- 2
- Cited by