Key Points
The incidence of acute respiratory distress syndrome rises with age and is likely related to the increased incidence of sepsis with increasing age.
Age-related changes in pulmonary function include a decrease in forced vital capacity (FVC), forced expiratory volume in 1 second (FEV1), and arterial partial pressure of oxygen (PaO2) and an increase in the oxygen arterial-alveolar (A–a) gradient.
Both the incidence of pulmonary embolism and the mortality from pulmonary embolism increase with age.
There are no specific guidelines related to optimal ventilator modalities for older patients.
For older patients with ventilator-associated pneumonia, aspiration or microaspiration often is a contributing factor.
Local hospital antibiograms should be used when treating patients with ventilator-associated pneumonia.
Introduction
Respiratory diseases in older patients are a major reason for admission to the intensive care unit (ICU), and critical complications related to underlying respiratory disease have a major impact on patient outcomes. The incidence of acute respiratory failure increases dramatically with age [Reference Behrendt1]. Older adults (defined as age > 65 years) comprise nearly 50 percent of ICU admissions in the United States [Reference Lerolle, Trinquart and Bornstain2,Reference Angus, Shorr and White3]. Among all ICU patients who receive mechanical ventilation, 125,000 survive to discharge. Half of these survivors are ultimately readmitted to the hospital, and 30 to 60 percent die within 6 months. Interestingly, there is also evidence that older adults are receiving more intense care in critical care unit settings, and this may be the cause of decreased mortality [Reference Lerolle, Trinquart and Bornstain2]. Therefore, understanding the issues related to older adults with respiratory problems is essential to delivering appropriate medical care and providing accurate prognostication for this population [Reference Wunsch, Guerra and Barnato4,Reference Kahn, Benson, Appleby, Carson and Iwashyna5].
Background: Respiratory Physiology and Age
The respiratory system undergoes many changes with aging that reduce reserve and have substantial impact on an individual’s capacity to tolerate the stresses of critical illness. Table 4.1 lists some of the age-related changes in respiratory physiology. Age-related changes in respiratory function are defined by decreased strength of the respiratory muscles, a decrease in the elastic recoil of the lung, and a decrease in chest wall compliance [Reference Janssens6]. Ventilatory reserve decreases substantially with age as a result of multiple interrelated physiologic changes: osteoporosis, kyphosis, and decreased mobility of joints (e.g., rib-vertebral articulation). Together these changes lead to decreases in lung volume and combined lung and chest wall compliance [Reference Sprung, Gajic and Warner7]. Elastance decreases, leading to increased lung compliance, as a result of changes in elastin structure and elastin-collagen ratios [Reference Knudson, Burrows and Lebowitz8,Reference Zeleznik9]. The combination of increased lung compliance and decreased chest wall compliance leaves total lung capacity unchanged, but the resulting increase in residual volume means that the vital capacity is reduced, along with a concomitant reduction in expiratory flow rates, as measure by forced expiratory volume in 1 second (FEV1) [Reference Knudson, Burrows and Lebowitz8]. There is also an age-related decrease in FEV1 of 10 to 30 ml per year and a concomitant decrease in diffusing capacity after the age of 40 [Reference Janssens, Pache and Nicod10,Reference Knudson, Kaltenborn, Knudson and Burrows11] (Figure 4.1). These limitations mean that the requirement for increased minute ventilation during exercise or illness is met in large part by increases in respiratory rate. Additionally, because age-related decreased chest wall compliance is proportionally larger than the increased lung compliance, the net compliance of the respiratory system is decreased, and therefore, resting work of breathing is increased and the diaphragm and abdominal muscles contribute proportionally more to the work of breathing than the thoracic muscles when compared with younger patients [Reference Turner, Mead and Wohl12].
Table 4.1 Respiratory System Age-Related Changes
Decreased vital capacity (VC) |
Decreased forced expiratory volume in 1 second (FEV1) |
Increased functional residual capacity (FRC) |
Increased residual volume (RV) |
Reduced elastic recoil of the lung |
Reduced arterial partial pressure of oxygen (PaO2) |
Increased O2 arterial-alveolar (A–a) gradient |
Increased chest wall stiffness, kyphoscoliosis |
Decreased respiratory muscle strength |
Decreased respiratory center sensitivity to hypoxia and hypercarbia |
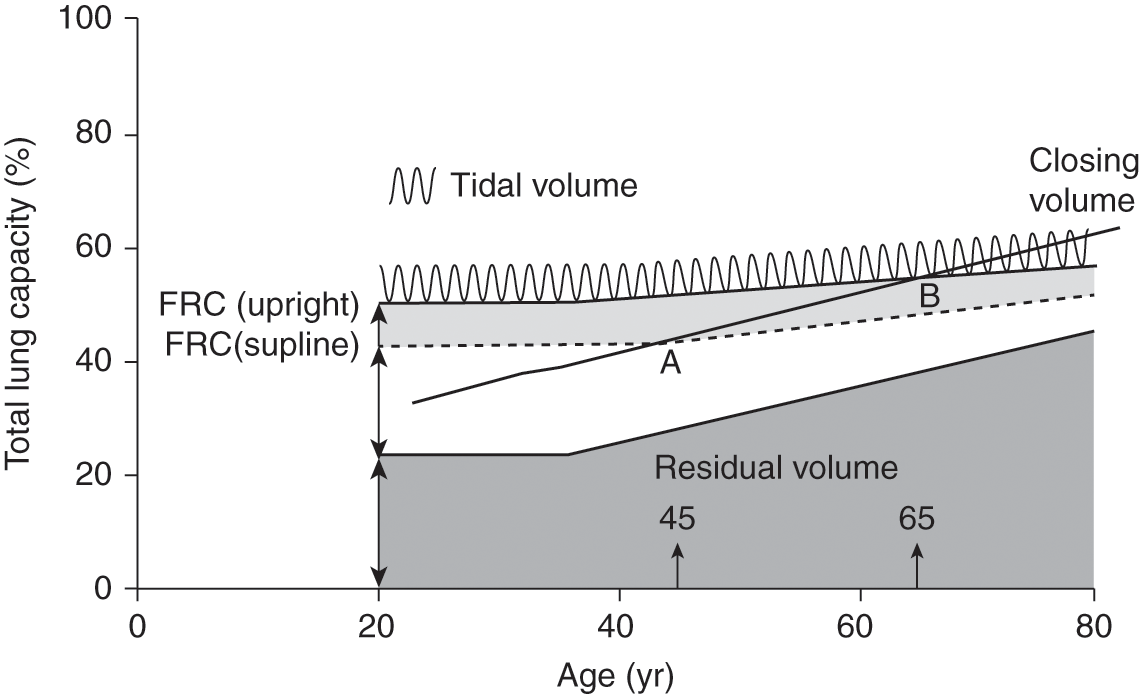
Figure 4.1 Changes in lung volumes and capacities with aging. Residual volume and functional residual capacity (FRC) increase with age, whereas total lung capacity remains the same. Closing volume increases with age and exceeds FRC in the supine position at about age 45 and exceeds FRC in the upright position at about age 65.
Respiratory muscle performance is impaired by age-related changes in muscle and bone relationships and increased FRC from decreased elastic recoil of the lung [Reference Turner, Mead and Wohl12]. Respiratory muscle status is related to nutritional status and lean body mass; therefore, malnutrition reduces muscle strength and maximal ventilation [Reference Arora and Rochester13]. Age is significantly correlated with reduced respiratory muscle function and walking capacity [Reference Watsford, Murphy and Pine14]. There is a progressive decline in diaphragmatic strength (25 percent) in healthy elderly persons. An investigation into the response of the respiratory muscles to graded exercise demonstrated greater participation of diaphragmatic motion together with rapid shallow breathing during lower-graded exercise in the elderly compared with the young [Reference Teramoto, Fukuchi, Nagase, Matsuse and Orimo15]. With regards to gas exchange, an increase in dead space and shunt fraction and a reduction in diffusing capacity have been associated with increasing age [Reference Stam, Hrachovina, Stijnen and Versprille16]. It is estimated that the arterial partial pressure of oxygen (PaO2) decreases by an average rate of 0.35 mmHg per year. Mean arterial oxygen tension on room air decreases from 95 mmHg at age 20 to less than 70 mmHg at age 80. These changes are caused by an increased degree of ventilation/perfusion (V/Q) mismatching and, to a lesser extent, pulmonary shunting. These changes would be expected to impair carbon dioxide elimination and oxygenation in the setting of a significant respiratory insult (e.g., acute respiratory distress syndrome [ARDS]). A study of airspace size relative to age found airspace enlargement in older subjects [Reference Gillooly and Lamb17]. In addition to the physiologic changes in lung function, the neural sensing and modulating responses by the central nervous system (CNS) of the respiratory system also change with age. Older subjects have a significantly lower ventilatory response to both hypoxia and hypercapnia, and occlusion-pressure response to both hypercapnia and hypoxemia is reduced by a similar magnitude. The alteration in CNS efferent control is thought to be secondary to the lower augmentation of respiratory drive in older adults [Reference Peterson, Pack, Silage and Fishman18].
Older adults are more likely to have concomitant illnesses that have an impact on their respiratory system. Congestive heart failure has a significant negative effect on respiratory muscle strength and tension-time index, which is important given that cardiac disease and respiratory disease are commonly coincident [Reference Mancini, Henson, LaManca and Levine19]. In one study, the prevalence of inspiratory muscle weakness was as high as 76 percent in patients who were hospitalized because of acute heart failure [Reference Verissimo, Casalaspo and Gonçalves20]. Hypertension, lower extremity edema, and diabetes are independently associated with a lower level of lung function [Reference Enright, Kronmal, Higgins, Schenker and Haponik21]. It is clear that concomitant diseases associated with aging taken together have a substantial impact on respiratory function.
Risk Factors for Respiratory Failure in Older Adults
Acute respiratory failure is a common complication of critical illness in older adults and is due in large part to the increase in prevalence of chronic illnesses, major organ dysfunction, and an increased risk of acquired causes of respiratory failure [Reference Marrie, Lau, Wheeler, Wong and Feagan22,Reference Ray, Birolleau and Lefort23]. The incidences of pulmonary embolism, chronic obstructive pulmonary disease (COPD), congestive heart failure, and community-acquired pneumonia all rise with age [Reference Marrie, Lau, Wheeler, Wong and Feagan22,Reference Rich24]. Furthermore, as many as 47 percent of patients over age 65 who have acute respiratory failure have two diagnoses as the etiology of their respiratory decompensation. This is in large part due to the high incidence of congestive heart failure and COPD in this population. The increased incidence of major organ dysfunction combined with decreased pulmonary reserve is likely responsible for the exponential increase in respiratory failure from the third through the ninth decades of life [Reference Behrendt1,Reference Siner and Pisani25,Reference El Solh and Ramadan26]. Specifically, in the surgical population, an investigation regarding risk factors for postoperative intubation demonstrated that smoking status, presence of COPD, emergent intervention, and age were important determinants of risk [Reference Alvarez, Samayoa-Mendez, Naglak, Yuschak and Murayama27]. A separate investigation had similar findings, confirming the role of age as a risk factor for unplanned postoperative intubations, and noted an 18-fold increase in mortality risk associated with unexpected requirement for mechanical ventilation [Reference Nafiu, Ramachandran and Ackwerh28]. In a review of surgical ICU admissions, it was noted that 48 percent of the readmissions were due to respiratory complications and that readmitted patients were older [Reference Timmers, Verhofstad, Moons and Leenen29].
Acute Pulmonary Embolism
The occurrence of pulmonary embolism (PE) increases with age, and elderly patients have the highest risk of venous thromboembolic disease (VTE), with an incidence greater than 1 percent per year [Reference Oger30]. With regard to the clinical presentation of PE, older adults report less pleuritic chest pain and are more likely to present with syncope [Reference Schouten, Geersing and Oudega31]. In an evaluation of older patients, it was noted that only 5 percent of those older than 80 years age have a negative D-dimer test compared with 50 percent of patients younger than 50 years of age [Reference Righini, Le Gal and Bounameaux32]. A multicenter prospective management outcome study of patients with suspected PE demonstrated that an age-adjusted D-dimer cutoff was associated with a larger number of patients in whom PE could be ruled out [Reference Righini, Van Es and Den Exter33].
Elderly patients with a PE have a high (>20 percent) 3-year mortality predominantly due to the high frequency of cancer and other comorbidities [Reference Faller, Limacher and Méan34]. Risk assessment is generally performed based on echocardiography and measurement of circulating biomarkers associated with right ventricular strain. While echocardiographic evidence of right ventricular dysfunction is often used clinically to stratify risk, the extent of right ventricular dysfunction does not appear to be associated with mortality in older adults [Reference Hofmann, Limacher and Méan35]. Cardiac biomarkers are more useful for identifying low-risk individuals with nonmassive PEs than echocardiography data, and these findings have been replicated in older adult populations [Reference Konstantinides and Goldhaber36,Reference Vuilleumier, Simona and Méan37].
New interventions for both massive and submassive PEs include ultrasound-assisted catheter-directed low-dose thrombolysis, which is still under investigation, but early studies have shown efficacy with possibly a lower risk of hemorrhage, although those studies may not be adequately powered to detect this complication [Reference Kucher, Boekstegers and Müller38,Reference Kuo, Banerjee and Kim39]. Meta-analyses of prior investigations of thrombolysis for massive PEs have suggested that bleeding complications, including intracranial hemorrhage, are higher in those with advanced age, but whether is it is age or simply the concomitant risk factors, including malignancy, that increase risk is unknown [Reference Chatterjee, Chakraborty and Weinberg40,Reference Fiumara, Kucher, Fanikos and Goldhaber41]. While there are no specific independent guidelines for elderly adults who are critically ill with massive PEs, the current literature suggests that they be treated similar to the general population, but with recognition of the potential for increased risk of complications due to age and burden of comorbidities.
Pneumonia in Older Adults
Several studies have demonstrated that older patients have an increased incidence of pneumonia and greater mortality after pneumonia compared with younger patients. The physiologic changes discussed earlier and a reduced ability to expectorate and clear bacteria and decreased physiologic reserve are factors that increase this risk. In addition, oropharyngeal bacterial colonization with Staphylococcus aureus, Klebsiella pneumonia, and Escherichia coli are more common in elderly patients and serve as harbingers of subsequent pneumonia. Patients with severe community-acquired pneumonia who are older than 65 years of age have many preexisting diseases, including COPD (48 percent), heart disease (16 percent), diabetes (18 percent), and malignancy (12 percent) [Reference Rello, Rodriguez, Jubert and Alvarez42]. Elderly patients are more at risk for aspiration, including silent aspiration [Reference Kikuchi, Watabe and Konno43]. Factors supporting this include alterations in the swallowing mechanism that occur with age, cognitive impairment, medication use, malnutrition, poor oral health, and Parkinson’s disease [Reference van der Maarel-Wierink, Vanobbergen, Bronkhorst, Schols and de Baat44].
Frail older patients may not present with the typical fever, cough, and shortness of breath that characterize pneumonia in younger patients. Many older patients with pneumonia present with nonproductive cough, delirium, anorexia, falls and dizziness, or signs of sepsis. Older patients who present with hypothermia and sepsis from pneumonia have significant in-hospital mortality [Reference Tiruvoipati, Ong and Gangopadhyay45].
While older patients frequently have bacterial pneumonia and sepsis precipitating an ICU admission, they also are frequently critically ill secondary to viral respiratory infections. Patients older than 65 years have higher rates of hospitalization for laboratory-confirmed influenza. In the United States during the 2014–15 influenza season, the rates of hospitalization for patients older than 65 years was 258 per 100,000 population compared with 41 per 100,000 population for younger patients [Reference D’Mello, Brammer and Blanton46].
A recent systematic review examined current evidence on antibiotic treatment of older adults with community-acquired pneumonia (CAP), healthcare-associated pneumonia (HCAP), hospital-acquired pneumonia (HAP), and ventilator-associated pneumonia (VAP). This study demonstrated that patients older than age 65 are often excluded from clinical trials of bacterial pneumonia, and no data were found on the comparative efficacy of antibiotic treatment in elderly adults compared with younger patients [Reference Avni, Shiver-Ofer and Leibovici47]. What the study did note was increased treatment failure rates in participants who were aged 65 years and older.
Ventilator-associated pneumonia is a complication for older patients in the ICU who are mechanically ventilated. There are no studies examining the recognition or treatment of VAP in older patients, and general guidelines should be followed. The 2016 Infectious Disease Society of America guidelines on HCAP and VAP make recommendations regarding treatment, including using hospital-specific antibiograms to guide empirical antibiotic choices. There are no recommendations based on patient age [Reference Kalil, Metersky and Klompas48]. One study using a molecular microbiology study of 44 patients age 60 years and older demonstrated that gastro-pulmonary aspiration is an important mechanism in the development of VAP, especially late-onset VAP [Reference Liu, Zhang and Lin49]. Risk for aspiration should be recognized and evaluated in all older patients on mechanical ventilation and after extubation.
Acute Respiratory Distress Syndrome in Older Adults
Acute respiratory distress syndrome (ARDS) is a disease resulting in acute hypoxemic respiratory failure most commonly due to sepsis, pneumonia, aspiration, trauma, pancreatitis, and transfusion of allogeneic blood products. The injury of ARDS involves the alveolar epithelium and the lung capillary endothelium. The definition of ARDS was revised (Berlin definition) in 2012 and demonstrates better predictive validity for mortality compared with the 1994 American-European Consensus Conference definition. Table 4.2 lists the Berlin definition of ARDS [Reference Ranieri, Rubenfeld and Thompson50]. The significantly increased incidence of ARDS associated with older age is partly due to the higher incidence of sepsis with aging, the primary risk factor for ARDS in adults [Reference Angus, Linde-Zwirble and Lidicker51,Reference Manzano, Yuste and Colmenero52]. An investigation of ARDS in the trauma population showed that the incidence of ARDS increases with age but plateaus in the 60- to 69-year-old population [Reference Hudson, Milberg, Anardi and Maunder53]. In a global incidence study, 10.4 percent patients with ICU admissions and 23.4 percent of patients requiring mechanical ventilation had ARDS. These results are similar to those of prior investigations, where clinical recognition of ARDS was only 51.3 percent for mild ARDS but increased to 78.5 percent for severe disease [Reference Bellani, Laffey and Pham54].
Table 4.2 Acute Respiratory Distress Syndrome: The Berlin Definition
|
Abbreviations: PaO2 = partial pressure of arterial oxygen; FIO2 = fraction of inspired oxygen; PEEP = positive end-expiratory pressure; CPAP = continuous positive airway pressure.
The first large randomized, controlled trial to evaluate a therapy for ARDS was published in 2000 and demonstrated that the ARDSnet approach to low-tidal-volume ventilation results in a substantial reduction in mortality [55]. The original ARDSnet investigation was notable for a young mean age and excluded patients with significant chronic lung disease, leaving open the question of whether this study is generalizable to the large population of older adults who develop sepsis and ARDS. Furthermore, because the low-tidal-volume strategy used tidal volumes and plateau pressures and older adults are known to have increased lung compliance and decreased chest wall compliance, it remains unknown whether the ARDSnet strategy would have the same effect in older adults with different pulmonary responses to similar volumes and pressures. A reanalysis of prior studies in ARDS found that while overall survival was high, older adults were twice as likely to die of acute lung injury and had greater difficulty achieving liberation from mechanical ventilation and being discharged from the ICU than younger adults [Reference Ely, Wheeler and Thompson56]. In addition to low-tidal-volume ventilation, investigators have examined several other therapies for ARDS. A follow-up study by the ARDSnet investigators examined the role of corticosteroids as adjunctive therapy and showed that pulmonary parameters improved but that the increased rate of muscle weakness led to an increase in reintubations, and this would be of even greater concern in an older adult population [Reference Steinberg, Hudson and Goodman57]. Prone positioning has been investigated several times over the past decade, and the most definitive study of prone positioning for ARDS demonstrated improved outcomes, and importantly, the average age was approximately 60 years, but with large standard deviations consistent with the fact that a fair number of these patients were older adults. This trial showed significant reductions in mortality and ICU length of stay, and this approach has been adopted as a component of standard care for those with ARDS [Reference Guérin, Reignier and Richard58].
Additional supportive therapies that have received great attention in the past decade include a resurgent interest in the use of extracorporeal membrane oxygenation (ECMO). Most institutions and protocols have generally excluded the use of ECMO in older patients (age greater than 60 or 65 years). The authors of the CESAR trial recommended transferring patients with severe but potentially reversible hypoxemic respiratory failure, based on Murray score, to an ECMO center to improve survival without disability. The study itself started with 766 patients, of which 586 were excluded, and of those excluded, 10 percent of those referred for enrollment were excluded due to age (>65 or <18 years). These age-based exclusions are fairly standard for ECMO, and thus the implications of ECMO for older adults are unclear [Reference Peek, Mugford and Tiruvoipati59]. In a retrospective review of the Extracorporeal Life Support Organization (ELSO) registry from 1990 to 2013 for adults of age greater than 65 years, survival for older adults was 41 percent versus 55 percent for the entire population. Nonsurvivors were more likely to have hemorrhagic complications (cannulation site, pulmonary hemorrhage, disseminated intravascular coagulation, excessive hemolysis while on ECMO support). Death from withdrawal of life support occurred in 11 percent of patients. While the mortality rate in this review was certainly higher for older adults, the survival rate was still in a range that many clinicians would consider acceptable for a lethal disease when there are limited alternative options [Reference Mendiratta, Tang and Collins60].
Mechanical Ventilation in Older Adults
There are no data to suggest that one mode of mechanical ventilation is better than any other mode of ventilation in older patients. As noted earlier in relation to ARDS, the study population used in the landmark study of low-tidal-volume ventilation did not include many older patients, and it also excluded many patients based on comorbidities, which are common in the elderly. Interestingly, a recent study using Medicare data demonstrated that among hospitalized nursing home residents with advanced dementia, there was an increase in the use of mechanical ventilation over time without substantial improvement in survival [Reference Teno, Gozalo and Khandelwal61]. The use of mechanical ventilation increased from 39 per 1,000 hospitalizations in 2000 to 78 per 1,000 hospitalizations in 2013 [Reference Teno, Gozalo and Khandelwal61]. As the number of ICU beds in a hospital increased over time, patients with advanced dementia were more likely to receive mechanical ventilation.
Discontinuation of Mechanical Ventilation and Prolonged Mechanical Ventilation
Expertise in liberation from mechanical ventilation has evolved over the past 20 years, yet it remains unclear specifically how the current knowledge applies to older adults. Because at baseline older adults have a respiratory exertional response that is similar to rapid shallow breathing, using this measure may be a less accurate marker of ongoing respiratory failure during weaning in the elderly [Reference Sprung, Gajic and Warner7,Reference Zaugg and Lucchinetti62]. Thus, physiologically, a patient may be judged to have failed a spontaneous breathing trial (SBT) when they have not. Yet this observation is further complicated by the fact that older adults also have a diminished response to hypoxia and hypercapnia, and therefore an older adult patient on a SBT may be more likely to appear comfortable despite having developed significant hypoxia or hypercapnia [Reference Peterson, Pack, Silage and Fishman18]. The most direct evidence regarding older adults and extubation comes from a study by Ely et al., who investigated outcomes of older patients in a large multicenter trial of ARDS [Reference Ely, Wheeler and Thompson56]. Although older patients achieved physiologic recovery from acute lung injury in equal proportions to younger patients, their ICU length of stay and duration of mechanical ventilation were increased because of higher reintubation rates. In a medical ICU population, El Solh et al. observed that patients older than age 70, compared with a younger matched cohort, were more likely to fail extubation because of an inability to handle secretions [Reference El Solh, Bhat, Gunen and Berbary63]. While liberation from mechanical ventilation in older adults remains an area in which there are limited data, it is clear from an understanding of physiology and our limited data that the intensivist should carefully consider the impact of aging on respiratory function when making decisions on liberation from ventilation.
Prolonged mechanical ventilation (PMV) is defined as requiring mechanical ventilator support for longer than 21 days. Approximately 300,000 individuals annually in the United States require mechanical ventilation in the acute setting for more than 4 days [Reference Zilberberg, Luippold, Sulsky and Shorr64]. Among these individuals, 3 to 7 percent will survive and remain ventilator dependent after 21 days [Reference MacIntyre, Epstein and Carson65]. Tracheostomy use in those requiring mechanical ventilation rose substantially from 1993 to 2008 (from 6.9 percent in 1993 to 9.8 percent) but then began a slow decline. This increase in the tracheostomy rate was driven primarily by patients in the surgical population and was associated with a decreased length of hospital stay [Reference Mehta, Syeda and Bajpayee66]. A study of 437 admissions to a long-term acute care hospital (LTACH) for PMV between 2001 and 2006 investigated the impact of age on survival and discontinuation of mechanical ventilation. Increasing age was associated with more physiologic abnormalities and comorbidities, but after adjustment for these factors, the authors did not find an independent effect of age on ability to wean [Reference Sansone, Frengley, Vecchione, Manogaram and Kaner67]. In the postacute settings, elderly adults were most likely to have successful discontinuation of mechanical ventilation if they had fewer comorbidities and less respiratory impairment, and interestingly, these factors were more important than physical function prior to the acute care hospitalization [Reference Dermot Frengley, Sansone, Shakya and Kaner68]. While the authors do not comment on this specifically, their data show that regardless of age, fewer than 10 percent of adults who failed to wean are alive at 24 months. While age alone was not associated with survival per se, for adults older than 84 years of age with PMV, those who were able to be weaned had a survival rate of only 20 percent at 24 months [Reference Dermot Frengley, Sansone, Shakya and Kaner68].
Conclusion
The respiratory system changes substantially with aging such that older adults have diminished ventilatory and oxygenation reserve and are at higher risk than younger adults of developing most common respiratory ailments, including pneumonia, ARDS, and respiratory failure. While much is known about these common respiratory ailments and there have been many controlled trials, age-specific knowledge is much more limited. Despite this, the evidence does suggest that while age increases the risk of developing certain adverse outcomes, it is important to keep in mind that it often the concomitant illness and comorbidities that drive the response to therapy and outcome more than simply the chronologic age.