Book contents
- Plant Evolutionary Developmental Biology
- Plant Evolutionary Developmental Biology
- Copyright page
- Contents
- Preface
- 1 Introducing Plant Evo-Devo
- 2 The Plant Phenospace
- 3 Tools
- 4 Genes and Genomes
- 5 Shoot and Root: Meristems and Branching
- 6 Leaves
- 7 Flowers and Fruits
- 8 Architecture and Syntax of the Plant Body
- 9 Pheno-Evo-Devo
- 10 Evolutionary Trends
- 11 Looking Ahead
- References
- Taxonomic Index
- Subject Index
- References
References
Published online by Cambridge University Press: 09 February 2018
- Plant Evolutionary Developmental Biology
- Plant Evolutionary Developmental Biology
- Copyright page
- Contents
- Preface
- 1 Introducing Plant Evo-Devo
- 2 The Plant Phenospace
- 3 Tools
- 4 Genes and Genomes
- 5 Shoot and Root: Meristems and Branching
- 6 Leaves
- 7 Flowers and Fruits
- 8 Architecture and Syntax of the Plant Body
- 9 Pheno-Evo-Devo
- 10 Evolutionary Trends
- 11 Looking Ahead
- References
- Taxonomic Index
- Subject Index
- References
Summary
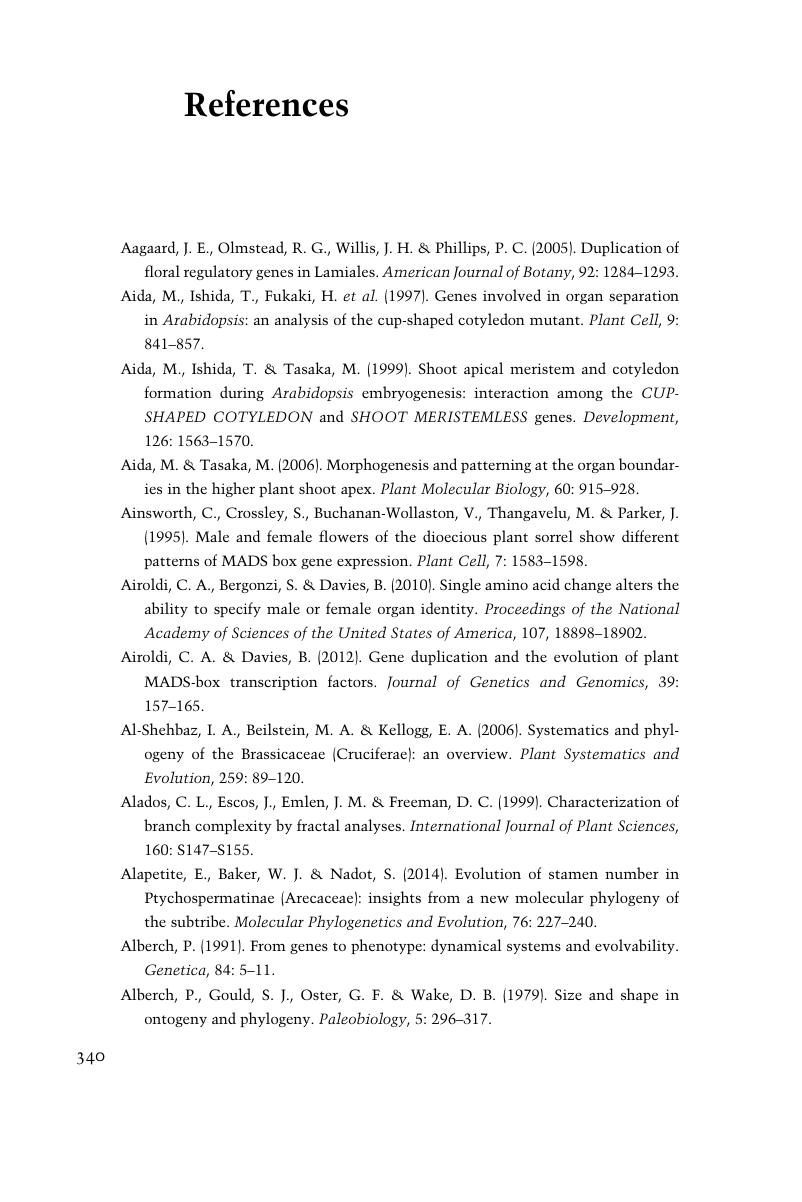
- Type
- Chapter
- Information
- Plant Evolutionary Developmental BiologyThe Evolvability of the Phenotype, pp. 340 - 435Publisher: Cambridge University PressPrint publication year: 2018